Exploring Chemical Polymers: Composition and Applications
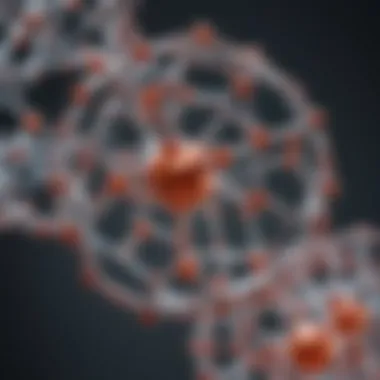
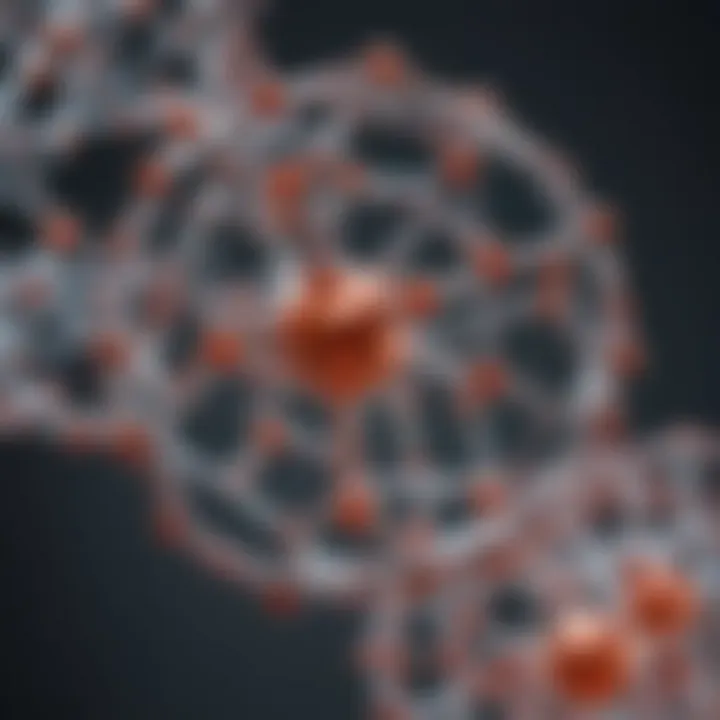
Research Overview
Chemical polymers are fundamental in a wide range of applications, impacting numerous industries including materials science, medicine, and electronics. This section examines the essential components that influence polymer behavior, as well as their multifaceted applications.
Summary of Key Findings
The exploration of chemical polymers reveals several significant insights:
- Molecular Structure: The arrangement of atoms in a polymer substantially affects its properties.
- Types of Polymers: Different classifications, such as thermoplastics and thermosets, have unique characteristics and uses.
- Synthesis Techniques: Processes such as addition polymerization and condensation polymerization play a crucial role in creating various polymers.
- Environmental Concerns: The ecological implications of polymer production and disposal are increasingly pressing, necessitating innovative recycling technologies.
Significance of Research
Understanding chemical polymers is vital for advancing technology and improving environmental sustainability. This research provides insights necessary for developing new materials and enhancing recycling methods, ultimately contributing to a more sustainable future.
Methodology
This section outlines the systematic approach used in exploring the attributes and impact of chemical polymers.
Research Design
The research design centers on a qualitative analysis of existing literature and experimental studies related to chemical polymers.
Data Collection Methods
Data were collected from reputable sources such as environmental studies, polymer synthesis protocols, and case analyses from various industries. Key methods of collection included:
- Literature Review: Critical analysis of existing research papers, articles, and reviews.
- Experimental Data: Insights from recent studies and laboratory experiments conducted on polymer materials.
Prologue to Chemical Polymers
Understanding chemical polymers is crucial for anyone engaged in the fields of materials science, chemistry, and engineering. These compounds form the basis of countless materials that we rely on in everyday life. Chemical polymers possess unique properties arising from their molecular structure, making them suitable for a wide range of applications. In this section, the importance of polymers will be examined through their definition, historical context, and practical implications.
Definition and Scope
Chemical polymers are large molecules composed of repeating structural units, known as monomers. These monomers link together through covalent bonds to create various polymeric structures. The scope of chemical polymers extends across various types, including natural and synthetic variations. The versatility of polymers allows them to be tailored for specific functions. For instance, they're employed in manufacturing plastics, fibers, and rubbers, as well as in advanced applications like biomedical devices.
The classification of polymers is essential to understanding their various uses. Polymers can be linear, branched, or cross-linked. Each structure imparts distinct properties that determine their function in products. Natural polymers, such as cellulose, have been used for centuries, while synthetic polymers, including polyethylene and polystyrene, have revolutionized material science in the 20th century. This diversity highlights the importance of studying both the inherent characteristics of polymers and their industrial applications.
Historical Context
The history of polymers traces back to natural substances used by ancient civilizations. For example, ancient Egyptians utilized natural rubber for various purposes. The scientific understanding of polymers began to evolve in the 19th century. In the early 1900s, scholars like Hermann Staudinger paved the way for modern polymer chemistry by proposing that polymers are macromolecules.
The synthetic polymer industry saw significant growth during and after World War II. The invention of nylon by Wallace Carothers marked the first major synthetic polymer. Subsequent developments led to the discovery of polyethylene by accident, which further transformed the materials landscape. Today, the production of polymers is a billion-dollar industry. Companies produce various types of plastics that are integrated into consumer goods, medical devices, and other essential products.
In summary, the study of chemical polymers provides insight into a fundamental aspect of modern materials. By understanding their definition, scope, and historical significance, we lay the groundwork for exploring their composition, properties, and applications.
Chemical Structure of Polymers
Understanding the chemical structure of polymers is essential in grasping their functionality and applications. The structure determines how polymers will behave under different conditions, influencing their durability, flexibility and suitability for specific uses. A detailed look at the elements that constitute this field reveals important aspects of polymers that affect everyday life and industrial processes.
Molecular Composition
Monomers
Monomers are the fundamental building blocks of polymers. Their unique chemical properties allow them to bond together, creating long chains through polymerization. The most notable characteristic of monomers is their ability to undergo various types of reactions to form different polymer types. For example, ethylene is a commonly used monomer in the creation of polyethylene, which is widely used in packaging.
The main benefit of focusing on monomers in this context is that their selection directly impacts the resulting polymer properties. For instance, using high-density polyethylene can lead to materials with significant tensile strength, making it a preferred choice in resilience-required applications. However, monomers can also contribute to environmental concerns due to their potential for toxicity, especially when discussing synthetic options.
Polymers Chains
Polymers chains refer to the actual long structures formed by linking monomers together. The arrangement and length of these chains are critical to determining the physical properties of the polymer. A key characteristic of polymer chains is their ability to create materials with varying densities and properties. This flexibility is extremely beneficial for tailored applications across industries.
The unique feature of polymer chains includes their diverse configurations, leading to either crystalline or amorphous structures. Crystalline structures provide strength and stability, while amorphous structures offer elasticity. Each type comes with its advantages and disadvantages. For instance, while crystalline polymers can withstand higher loads, they may be less flexible compared to their amorphous counterparts.
Polymeric Architecture
Linear Polymers
Linear polymers are composed of long, unbranched chains of monomers. Their streamlined structure contributes to a series of beneficial properties, particularly in terms of tensile strength and clarity. This type of polymer is often popular in creating high-strength materials, making them a good choice in fabrics and films.
The unique feature of linear polymers lies in their ability to pack closely together, which enhances their strength. However, this structure also makes them harder to process under certain conditions, which can pose challenges in manufacturing processes.
Branched Polymers
Branched polymers are distinguished by side chains that extend from the main polymer backbone. These polymers have gained attention for their unique characteristics, such as increased solubility and lower density compared to linear polymers. They are often utilized in applications where flexibility is a priority, such as in adhesives and coatings.
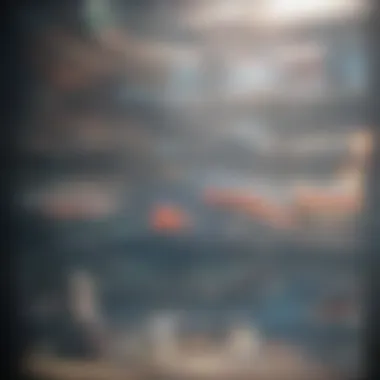
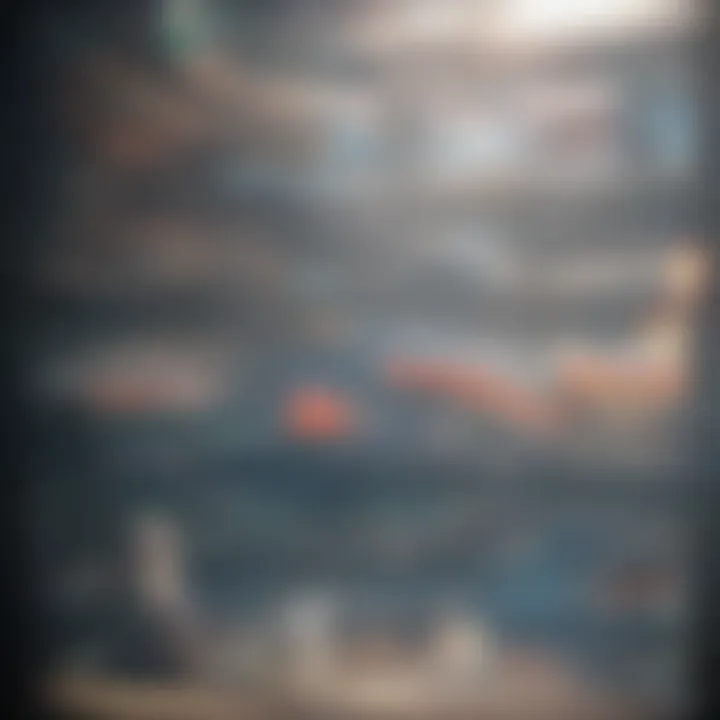
Despite the benefits, branched polymers can have reduced tensile strength compared to their linear counterparts. This makes them unsuitable for applications requiring high mechanical stress, but their versatility allows for innovative use in diverse materials.
Cross-linked Polymers
Cross-linked polymers are composed of chains that are connected to one another through covalent bonds. This architecture creates a three-dimensional network that greatly enhances the durability of the material. A key characteristic to note is the exceptional heat resistance and mechanical strength offered by cross-linked polymers. They are often favored in applications involving heavy-duty requirements, such as insulation and coatings.
The unique feature of cross-linked polymers is their ability to maintain structural integrity under extreme conditions. Nevertheless, the rigid structure might be less advantageous where flexibility is needed, limiting their use in some applications.
"The arrangement of polymer chains not only dictates their strength and flexibility but also their responsiveness to environmental factors."
In summary, the chemical structure of polymers is crucial for determining their functional attributes. By understanding the components and characteristics of monomers, polymer chains, and architectural types such as linear, branched, and cross-linked, we gain valuable insights into their applications and implications across various fields.
Types of Chemical Polymers
The classification of chemical polymers is essential for understanding their diverse properties and applications. This section explores different types of polymers, ranging from natural to synthetic, discussing their characteristics and significance in various industries. Knowing these types allows researchers and professionals to select appropriate materials for specific uses, addressing the needs of modern applications.
Natural Polymers
Examples and Occurrences
Natural polymers are organic materials found in nature. They occur in a variety of forms, such as proteins, polysaccharides, and nucleic acids. Examples include cellulose, which makes up the cell walls of plants, and chitin, found in the exoskeletons of insects and crustaceans. These polymers play crucial roles in biological processes.
One key characteristic of natural polymers is their molecular harmony with living systems. They are often biocompatible and can inherently provide functionality in biological contexts. Furthermore, natural polymers are frequently employed in the food industry and medical fields, primarily due to their safety and sustainability features. The unique feature of these polymers’s ability to biodegrade offers advantages for environment-sensitive applications. However, sourcing these materials can sometimes raise ethical and sustainability concerns, especially in cases where overharvesting occurs.
Biodegradability
Biodegradability is a major feature of natural polymers. This characteristic allows them to decompose naturally through biological processes, reducing environmental impact. Unlike synthetic polymers, which can linger in ecosystems for hundreds of years, natural polymers break down under suitable conditions, making them a more favorable option in the context of pollution.
This property is especially critical given current concerns about plastic waste, as using biodegradable options can alleviate some ecological burdens. However, biodegradability does come with limitations. For example, under certain conditions, some natural polymers may decompose too quickly, reducing their lifespan in practical applications. This necessitates considerations on how to effectively utilize their properties in both commercial and environmental contexts.
Synthetic Polymers
Production Methods
Synthetic polymers are artificially created through chemical processes. The main methods of production include addition polymerization and condensation polymerization. These processes enable the formation of a wide range of materials, tailored for specific applications.
A key characteristic of synthetic polymers is their versatility in production. This allows for the customization of properties such as strength, flexibility, and resistance to chemicals, making them suitable for various industries, including automotive and electronics. The unique aspect of production methods enables the large-scale manufacturing of materials, which results in cost-efficient options for consumers. However, the environmental concerns related to the production processes, including resource consumption and emissions, spotlight the need for sustainable practices.
Common Applications
Synthetic polymers find applications across numerous fields, including textiles, packaging, and healthcare. For instance, polyethylene is widely used in packaging materials due to its durability and flexibility. In the medical field, polylactic acid is used for its biocompatibility in sutures and drug delivery systems.
A major strength of these synthetic materials is their ability to be formed into complex shapes and structures, enabling innovation in product design. However, the reliance on fossil fuels for production can create sustainability challenges, mandating a shift toward alternative processes or materials. Understanding these applications supports ongoing research into developing improved synthetic options that align with global sustainability goals.
Synthesis of Chemical Polymers
The synthesis of chemical polymers is a cornerstone of modern materials science. It involves the transformation of small molecules, known as monomers, into larger, more complex structures called polymers. Understanding this process is essential to grasp how polymers achieve their unique properties and applications across various industries. The choice of polymerization method directly impacts the characteristics of the resultant polymers.
Polymerization Techniques
The synthesis of polymers can primarily be categorized into two techniques: addition polymerization and condensation polymerization. Each method has its distinct mechanisms and outcomes.
Addition Polymerization
Addition polymerization is notable for producing polymers without the generation of by-products. The key characteristic of this process is that it involves the opening of unsaturated bonds, typically double bonds in the monomers. This property makes addition polymerization a beneficial method for creating various plastics, such as polyethylene and polystyrene.
A unique feature of addition polymerization is its ability to create high molecular weight polymers quickly. This rapid production rate enhances its appeal in industrial applications. Nevertheless, one disadvantage is that the reactions can sometimes require precise conditions and specific initiators, making the process more complex than some alternatives.
Condensation Polymerization
Condensation polymerization, in contrast, involves the creation of polymer chains through stepwise reactions, where each step results in the release of small molecules, commonly water. This process is significant because it allows for the synthesis of diverse polymer types, including polyesters and polyamides.
The hallmark of condensation polymerization is its versatility in producing both linear and branched polymers. However, the release of by-products can complicate the purification stage, which can be seen as a disadvantage in certain applications. Despite this, the process often results in polymers with specific properties that are highly sought after in specialized applications.
Factors Influencing Polymerization
Several factors influence the efficiency and outcomes of polymerization processes, including temperature, pressure, and catalysts. Each factor plays a crucial role in determining the success of polymer synthesis.
Temperature
Temperature is a critical factor in polymer synthesis. It affects reaction rates and can determine the overall yield of the desired polymer. Generally, higher temperatures speed up reactions, but they can also lead to unwanted side reactions. Finding the optimal temperature is essential to maintain control over the polymerization process. A significant benefit of adjusting temperature is its influence on polymer characteristics, notably molecular weight. However, caution is needed, as excessively high temperatures may degrade the monomers or the formed polymers.
Pressure
Pressure also plays an important role, especially in reactions where gases are involved. Higher pressures can increase the reaction rate and yield of the desired polymer. This is especially useful in the production of polymers that require gas-phase reactants. However, managing pressure can be challenging and may require specialized equipment, which adds to production costs.
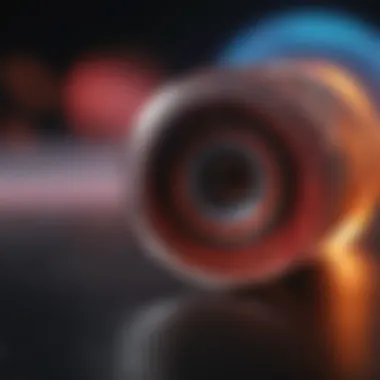
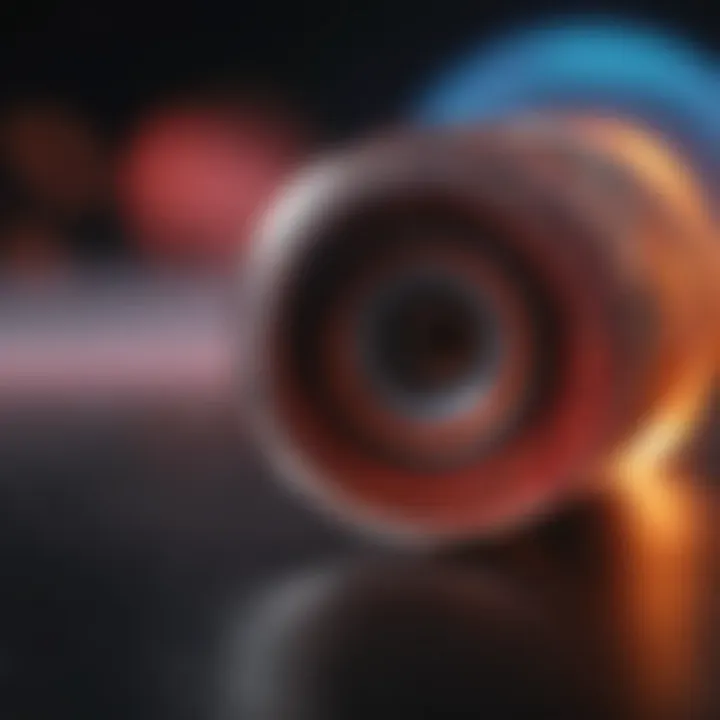
Catalysts
The use of catalysts in polymer synthesis is another crucial factor. Catalysts lower the activation energy required for a reaction, enhancing the rate of polymerization. They can significantly improve the efficiency of both addition and condensation methods. A unique advantage of using catalysts is their ability to enable reactions under milder conditions, thus preserving sensitive monomers or formed polymers. However, the selection and performance of catalysts can vary, leading to differences in polymer properties.
Catalysts are essential in developing specific polymer characteristics, enabling controlled reactions and reduced energy use.
Understanding the interplay of these factors is vital for researchers and manufacturers aiming to optimize polymerization processes for desired outcomes.
Properties of Chemical Polymers
Understanding the properties of chemical polymers is crucial for several reasons. These properties define how polymers behave under different conditions and influence their applications in various industries. Knowledge of both mechanical and thermal properties is fundamental to their utilization in product design, safety standards, and efficiency of performance. For example, knowing how a polymer responds to stress or changes in temperature can help in selecting the appropriate material for medical devices or packaging.
The exploration of these properties also reveals the versatility of polymers. They can be engineered for specific tasks through modifications of their chemical structure, which directly affects their mechanical and thermal properties. This adaptability makes them suitable for a wide range of applications, from construction materials to cutting-edge medical devices.
Ultimately, a comprehensive understanding of polymer properties enables manufacturers, researchers, and engineers to innovate new materials that meet specific needs while ensuring optimal performance and sustainability.
Mechanical Properties
Mechanical properties are a key aspect of how polymers function in practical scenarios. These properties include elasticity and tensile strength, both of which greatly influence the durability and usability of polymer products.
Elasticity
Elasticity refers to a polymer's ability to return to its original shape after being stretched or deformed. This characteristic is particularly important in applications where flexibility and resilience are required. The key characteristic of elasticity is the material's capacity to absorb energy and distribute stress. It is a popular choice for products like rubber bands, which benefit from their ability to stretch and return without damage.
A unique feature of elasticity in polymers is the hysteresis effect, where energy is lost in the form of heat during the deformation cycle. This can be advantageous in applications like damping systems, where energy absorption is desired. However, excessive elasticity may lead to energy loss, which could be a disadvantage in high-performance applications.
Tensile Strength
Tensile strength is the measure of a material's ability to withstand pulling forces without breaking. This property is essential for applications where structural integrity is critical. The key characteristic of tensile strength lies in its capacity to indicate how much load a polymer can bear before failure. It is an important aspect for materials used in construction and manufacturing, ensuring safety and reliability.
A notable feature of tensile strength in polymers is that it can vary greatly based on the polymer composition and structure. Generally, stronger polymers like Kevlar are used in armor and safety gear due to their high tensile strength. However, some polymers may be less strong and more suitable for lightweight applications where strength is less critical.
Thermal Properties
Thermal properties of polymers, including glass transition temperature and thermal conductivity, are crucial for understanding how polymers respond to heat. These properties are significant when considering their long-term performance and stability in varying environmental conditions.
Glass Transition Temperature
The glass transition temperature (Tg) represents the temperature range over which a polymer transitions from a hard and glassy state to a softer, more rubber-like state. A key characteristic of Tg is its influence on the mechanical properties of polymers as they heat or cool. This property is often considered when designing products meant to function in fluctuating temperature environments.
The unique feature of glass transition temperature is that it can significantly impact a polymer's usability range. If a polymer operates above its Tg, it may become too soft and lose structural integrity. Conversely, operating below the Tg could lead to brittleness. Balancing this temperature range is vital for products requiring consistent performance, such as adhesives and coatings.
Thermal Conductivity
Thermal conductivity determines how well a polymer can conduct heat. This property is particularly important in applications where heat management is required, like in electronics and thermal insulation materials. The key characteristic of thermal conductivity involves its ability to transfer heat efficiently. Polymers with low thermal conductivity are often used as insulators, while those with higher conductivity may be used in heat dissipation applications.
A unique feature of thermal conductivity in polymers is that it can be altered by adding fillers or modifying the polymer structure. For instance, polymers containing metal fillers can significantly enhance their thermal conductivity. However, this could impact other properties, like mechanical strength, necessitating careful consideration during material selection.
Ending
The mechanical and thermal properties of chemical polymers are foundational for their application in industry. Understanding these aspects enables informed decisions in material selection and product design, creating products that perform efficiently and sustainably. The adaptability of polymers makes them a key material in innovative applications, benefiting a wide range of sectors.
Applications of Chemical Polymers
The applications of chemical polymers are vast and varied, spanning numerous industries and sectors. Understanding these applications is crucial, as polymers play an integral role in both everyday products and advanced technologies. From packaging materials to medical devices, the significance of polymers cannot be understated. Their properties can be tailored to fit specific requirements, making them essential in enhancing functionality and performance. Furthermore, this section will delve into various aspects, including industry applications and medical use cases, highlighting both benefits and critical considerations.
Industry Applications
Packaging
Packaging is one of the most important applications of chemical polymers. The demand for durable and lightweight materials has led to the widespread use of polymers in packaging designs. Common polymers used include polyethylene, polypropylene, and polystyrene, which contribute to both the preservation of products and convenience for consumers. The key characteristic of polymer packaging is its ability to be customized -- various thicknesses and barriers can be created to suit different products.
However, the main advantage of using polymers in packaging is their versatility: they can be molded into various shapes and sizes. This adaptability makes them popular in food, electronics, and cosmetics sectors. Nevertheless, we must also consider the environmental impacts associated with plastic waste. Recycling and sustainability initiatives are becoming increasingly important as consumer awareness grows.
Construction Materials
Chemical polymers also find significant roles in construction materials. Polymers—like polyvinyl chloride (PVC) and polyurethane—are used extensively in pipes, siding, and insulation. The unique properties of these materials, such as water resistance and thermal insulation, contribute to building longevity and energy efficiency. Construction materials made of polymers can withstand harsh environmental conditions.
The primary benefit of polymer-based construction supplies is their durability and lightweight nature. This makes transport easier and can reduce overall construction costs. However, certain polymers may require special considerations in their production and disposal due to environmental concerns. As a result, innovation in sustainable alternatives is essential.
Medical Applications
Drug Delivery Systems
In the realm of medicine, polymers play a pivotal role in drug delivery systems. These systems are designed to release pharmaceutical compounds in a controlled manner, allowing for targeted therapy and improved patient outcomes. Polymers can be engineered to form hydrogels or nanoparticles, which encapsulate drugs and release them gradually. This precise delivery can minimize side effects and enhance drug efficacy.
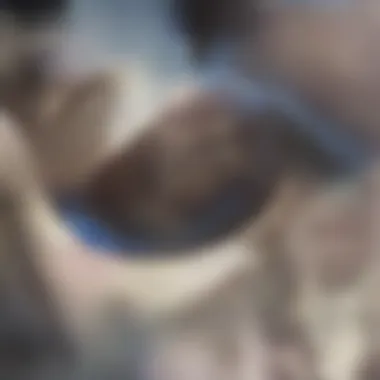
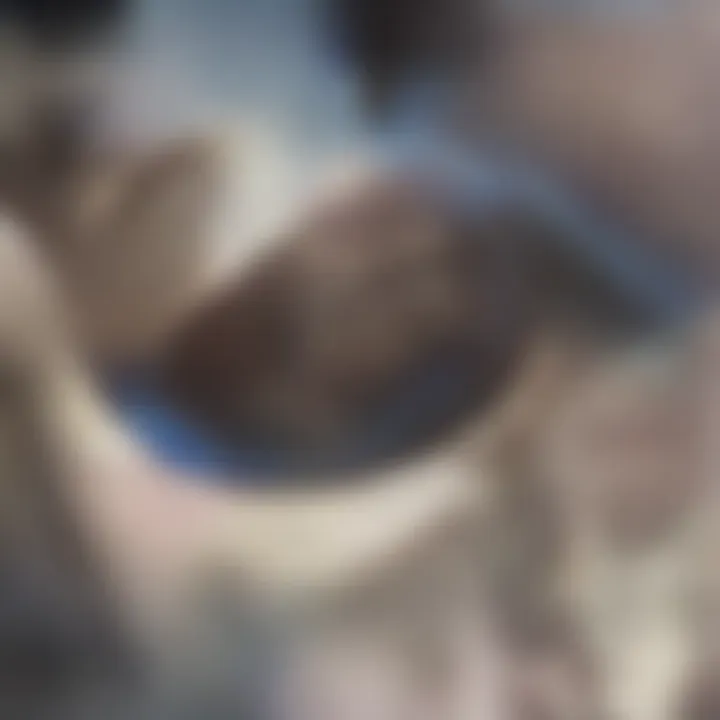
The key characteristic of drug delivery systems lies in their biocompatibility, which ensures that the polymers do not interfere with the body’s natural processes. This makes them a beneficial choice in contemporary medicine. However, while innovative, challenges exist regarding regulatory approval, as long-term effects of polymer interactions in the body are still under research.
Biomedical Devices
Biomedical devices are another crucial application of chemical polymers. They encompass a wide range of products, including prosthetics, implants, and diagnostic devices. Many of these devices are composed of biodegradable polymers, which can dissolve after serving their purpose, reducing the need for additional surgeries.
The most notable characteristic of biomedical devices is their functionality combined with compatibility with biological systems. This unique feature helps in promoting effective healing and integration with surrounding tissues. Despite the benefits, careful attention must be given to material selection and manufacturing processes to avoid complications like inflammation or rejection.
In summary, the applications of chemical polymers are comprehensive and critical for various industries, especially in packaging, construction, and healthcare. As research continues, the ability to improve and innovate these materials remains a priority for future developments.
Environmental Considerations
Environmental considerations play a crucial role in the discourse surrounding chemical polymers. As the use of these substances continues to proliferate in various applications, understanding their impact on the environment is more necessary than ever. The increasing presence of plastic pollution and the depletion of natural resources are just two major issues that arise from polymer usage. These concerns not only affect ecosystems but also challenge sustainability and human health. Therefore, focusing on the environmental aspects provides insight into potential solutions and practices aimed at mitigating negative impacts associated with polymers.
Impact of Chemical Polymers
Plastic Pollution
Plastic pollution has emerged as a significant environmental concern, directly tied to the widespread use of chemical polymers, particularly in consumer goods. Plastic products are typically non-biodegradable, leading to their accumulation in landfills and oceans. This pollution disrupts marine life and ecosystems, making it a prominent topic in environmental studies. In this article, plastic pollution is critically analyzed, shedding light on its key characteristics and implications.
One characteristic of plastic pollution is its longevity in the environment. Unlike organic materials, plastics can persist for hundreds of years. This unique feature creates long-term environmental challenges. On one hand, plastics offer durable packaging solutions in industries; on the other hand, their disposal issues and the persistent nature invoke significant concerns regarding waste management.
Resource Depletion
Resource depletion, often related to extracting materials for polymer production, is another pressing issue. The manufacturing of chemical polymers relies heavily on petroleum-based resources. As these finite resources diminish due to over-extraction, industries face heightened scrutiny. This article emphasizes the importance of understanding resource depletion as it directly frames the conversation around sustainability in polymer production.
A key characteristic of resource depletion is its impact on economies. Reduced accessibility to raw materials can lead to increased prices and competition among industries. This notion raises awareness of the urgent need for innovative solutions. The unique feature of resource depletion lies in its intertwined relationship with environmental degradation and economic stability, presenting both challenges and opportunities for alternative practices.
Sustainable Practices
Bio-based Polymers
Bio-based polymers represent an evolving solution to the problems associated with chemical polymers. Sourcing from renewable biological resources, these polymers showcase a sustainable alternative to conventional plastics. Their production relies on materials such as corn starch, sugarcane, and other plant derivatives. This article discusses how the adoption of bio-based polymers can potentially reduce environmental impact.
One important aspect of bio-based polymers is their ability to be sustainably sourced. The utilization of renewable resources lessens dependency on fossil fuels, contributing to fewer carbon emissions during production. However, they are not without downsides. Some bio-based polymers may still have limitations in terms of usability compared to their synthetic counterparts, and this aspect needs continuous research.
Recycling Technologies
Recycling technologies are critical in addressing the challenges posed by chemical polymers. Effective recycling can significantly lessen the amount of waste and reduce the demand for virgin materials. This article delves into various advanced recycling methods that are reshaping how polymers are processed at the end of their lifecycle.
A key characteristic of recycling technologies is their capacity to transform waste into usable products. Technologies such as mechanical recycling and chemical recycling demonstrate the potential to repurpose materials, thereby mitigating issues of plastic pollution. However, not all polymers are equally recyclable. The unique feature here lies in the complexity of polymer structures, sometimes impeding efficient recycling. Understanding these limitations is pivotal for advancing recycling efforts.
Future Directions in Polymer Research
Research into chemical polymers is an ongoing endeavor that has far-reaching implications for various fields. The future directions in polymer research are crucial for enhancing existing materials and developing new ones that can better meet the demands of contemporary society. This section explores this vital area, focusing on innovative techniques and interdisciplinary applications. The advancements in polymer science not only aim to improve the functionality and sustainability of polymers but also address some of the pressing challenges related to environmental concerns.
Innovative Polymerization Techniques
Green Chemistry Approaches
Green chemistry approaches are becoming increasingly important in the field of polymer research. These methods prioritize sustainability by minimizing the use of hazardous substances and reducing waste. The main characteristic of green chemistry approaches is their focus on using renewable resources and energy-efficient practices. They significantly contribute to reducing the ecological footprint of polymer production.
One of the unique features of these approaches is their emphasis on non-toxic reactants. This characteristic allows for safer working conditions and reduces the harmful impacts of chemical processes on the environment. Green chemistry is beneficial because it fosters the development of biodegradable polymers, promoting a circular economy. However, there are some challenges. The initial costs for implementing these methods can be high, and the scaling up of production processes may present difficulties.
Nanotechnology in Polymer Science
Nanotechnology plays a pivotal role in advancing polymer science. This field involves manipulating materials at the molecular and atomic levels to create new functionalities. A key characteristic of nanotechnology in polymer research is its potential to enhance the properties of polymers, such as strength, durability, and responsiveness to stimuli.
The unique feature of nanotechnology is its ability to improve interaction between polymers and other materials, resulting in composites that perform better than traditional counterparts. These advancements in functionality make nanotechnology an attractive choice for various applications, such as aerospace and healthcare. Despite its importance, challenges exist regarding the long-term safety and environmental impact of nanomaterials, which require thorough investigation.
Interdisciplinary Applications
Smart Materials
Smart materials are at the forefront of polymer research and application. These materials can respond to external stimuli such as heat, light, or pressure. Their ability to change properties makes them valuable in various fields, including robotics, automotive, and medicine. The adaptability of smart materials allows for innovations like self-healing surfaces and color-changing fabrics.
The unique feature of smart materials is their transformation capability, which can be tailored for specific applications. Their broad applicability in different sectors marks them as a popular choice among researchers and industries alike. Nonetheless, the complexity of designing these materials can pose significant challenges in terms of production and testing.
Energy Storage Solutions
Energy storage solutions are becoming increasingly important as the demand for sustainable energy rises. Polymers play a critical role in this field, especially in the development of batteries and supercapacitors. The key characteristic of polymer-based energy storage systems is their lightweight and flexible nature, which facilitates integration into various devices. They are particularly beneficial for portable electronics and electric vehicles.
A unique feature of polymer energy storage solutions is their ability to provide efficient energy delivery and management. Despite their advantages, issues related to the longevity and performance of these polymer systems may limit their applications. Research aimed at overcoming these challenges is essential to fully realize their potential in the growing energy landscape.
"Advancements in polymer research not only enhance material properties but also address urgent environmental and energy concerns, shaping the future of technology."
Ending
The exploration of chemical polymers brings into focus their vital role in numerous fields, underscoring their significance in contemporary material science and technology. This article highlighted several key aspects of polymers, including their compositions, properties, and a wide array of applications. Importantly, we discussed the innovative synthesis techniques that facilitate polymer creation and the environmental implications of their usage.
Understanding the chemical structure of polymers is crucial as it directly influences their mechanical and thermal properties. These properties determine their utility in various industries, from packaging to biomedical devices. In recent years, research has shown that advancing polymerization techniques can lead to more effective methods of production, contributing to enhanced material performance. For the environment, the conversation has shifted towards sustainability—where bio-based polymers and recycling technologies are becoming increasingly relevant.
"The future of polymers is not just in their use, but also in how we manage their lifecycle to ensure ecological balance."