Building a Sustainable Hydrogen Fuel Cell Guide
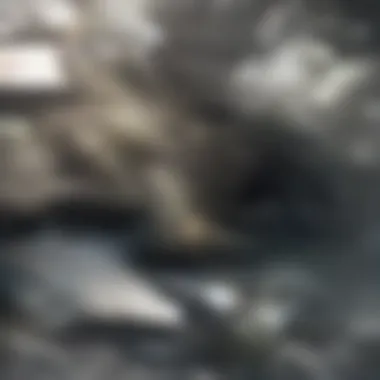
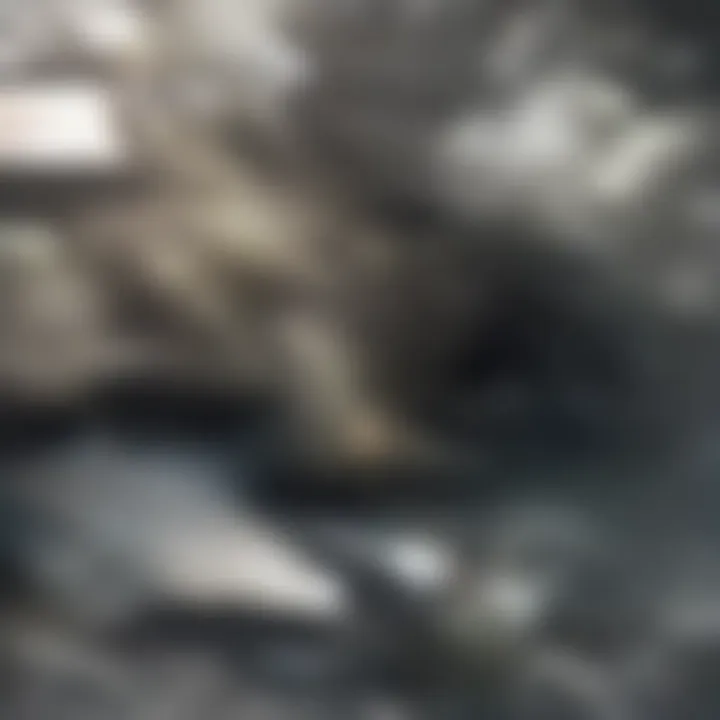
Intro
Hydrogen fuel cells are receiving attention for their potential role in sustainable energy production. These systems transform chemical energy into electrical energy with impressive efficiency and minimal environmental impact. Understanding how to create hydrogen fuel cells can aid students, researchers, and engineers in harnessing this technology. This section sets the stage for an in-depth exploration of the construction process, as well as the fundamental concepts that underline the operation of hydrogen fuel cells.
Research Overview
Summary of Key Findings
Numerous studies have highlighted the advantages of hydrogen fuel cells. Research indicates that they can produce power with greater efficiency than conventional combustion engines, leading to reduced emissions of harmful pollutants. Moreover, hydrogen, when derived from renewable sources, offers a way to store energy. This makes hydrogen fuel cells an innovative solution to the increasing demand for clean energy.
Significance of Research
The significance of this research extends beyond environmental concerns. Hydrogen fuel cells represent a pathway toward energy independence. They can power a variety of applications from transportation to stationary energy sources. Furthermore, developments in fuel cell technology can stimulate economic growth by creating jobs in the renewable energy sector. Therefore, a deeper understanding of fuel cell assembly is crucial for advancing this promising technology.
Methodology
Research Design
The methodology employed in the construction of hydrogen fuel cells will rely on both theoretical and practical approaches. The theoretical component includes studying the chemical and physical principles involved, while the practical aspect encompasses the assembly and operational testing of various types of fuel cells.
Data Collection Methods
Data will be gathered through a combination of experimental results and literature reviews. Experiments will be conducted in controlled environments, focusing on the measurement of efficiency, power output, and reaction rates. Additionally, technical specifications from existing hydrogen fuel cell systems will be analyzed. The goal is to compile a comprehensive knowledge base that supports the practical application of hydrogen fuel cells in real-world situations.
Prelims to Hydrogen Fuel Cells
The exploration of hydrogen fuel cells is significant in today’s quest for sustainable energy systems. Fuel cells are viewed as a crucial technology for reducing greenhouse gas emissions and reliance on fossil fuels. As we transition toward more environmentally friendly energy solutions, understanding hydrogen fuel cells becomes imperative for researchers, engineers, and students alike.
Hydrogen fuel cells operate on a simple principle, utilizing the chemical reactions between hydrogen and oxygen to generate electricity, water, and heat. The importance of this technology lies in its potential to provide clean energy and its adaptability across various applications: from powering vehicles to supplying electricity for homes and industries.
Moreover, hydrogen as a fuel source presents several benefits. It is abundant, can be produced from various feedstocks, and, when used in fuel cells, emits only water vapor as a byproduct. This characteristic makes it appealing from both an environmental and a performance standpoint. Additionally, hydrogen fuel cells support energy storage and distribution in ways that traditional batteries cannot, helping in load balancing in electrical grids.
The construction and implementation of hydrogen fuel cells involve notable technical considerations. Various components need to work seamlessly together, requiring precision in design and assembly. Thus, the objective of this article is to offer a comprehensive guide, focusing on the essential elements of construction, necessary materials, and practical insights for optimizing performance.
"The future of energy is hydrogen, unlocking sustainability for various industries."
Through this article, we aim to provide clarity and depth in understanding hydrogen fuel cells, ensuring that readers have a firm grasp of both foundational concepts and advanced construct strategies. This knowledge serves as a foundation for further innovation in the field of sustainable energy technologies.
Principles of Operation
Understanding the principles of operation for hydrogen fuel cells is crucial for anyone interested in constructing these systems. This section explains the essential chemical reactions that drive fuel cells and the mechanics of how energy conversion occurs. Grasping these fundamental concepts helps in optimizing performance and troubleshooting potential issues. Moreover, it lays a solid foundation for advanced research and practical applications in fuel cell technology.
Chemical Reactions
At the heart of hydrogen fuel cell operation is the electrochemical reaction between hydrogen and oxygen. The reaction can be summarized with the equation:
[
2H_2 + O_2 \rightarrow 2H_2O + \textenergy
]
This process takes place within the fuel cell, where hydrogen gas is supplied to the anode and oxygen is supplied to the cathode. When hydrogen molecules reach the anode, they undergo a reaction facilitated by catalysts, typically platinum. This reaction splits hydrogen molecules into protons (H⁺) and electrons (e⁻).
The separation of protons and electrons is pivotal. The protons pass through the electrolyte membrane, while the electrons take an external circuit, creating an electric current. At the cathode, oxygen reacts with the protons that have traversed the membrane and the electrons arriving from the external circuit. This entire process results in water and heat as byproducts, making it a clean and sustainable energy source. The efficiency of this reaction can greatly affect the overall performance of the fuel cell.
Energy Conversion Mechanics
The energy conversion mechanics of hydrogen fuel cells involve transforming chemical energy into electrical energy. This conversion is characterized by high efficiency compared to traditional combustion processes. The overall efficiency of fuel cells can reach up to 60%, and with the utilization of waste heat in combined heat and power systems, this value can be higher.
The mechanics begin when the flow of electrons through the external circuit generates electrical power. This power can be used directly to drive motors, power electronics, or generate heat.
Key factors influencing energy conversion include:
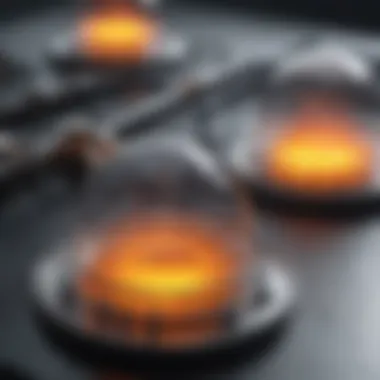
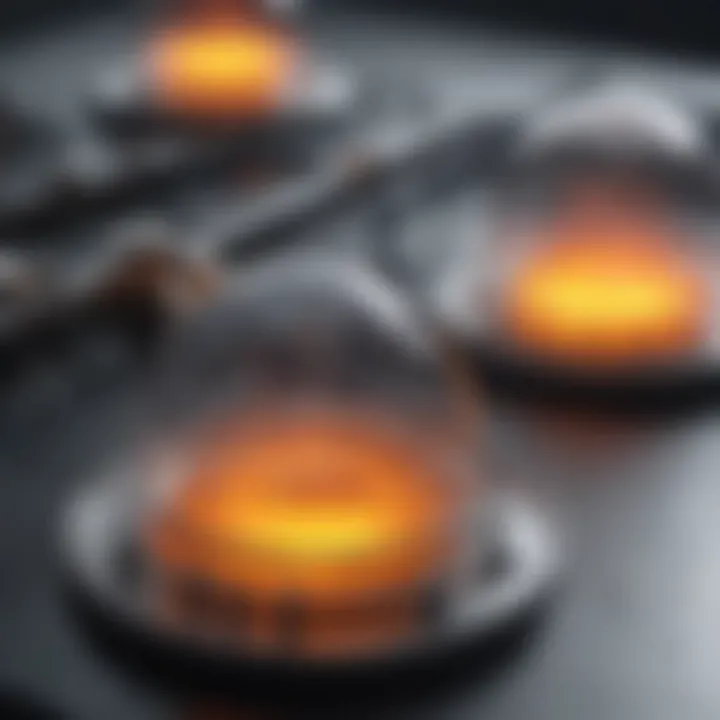
- Temperature: Higher temperatures can increase reaction rates but may also impact the lifespan of components.
- Pressure: Elevated pressure on hydrogen gas can improve performance and energy output.
- Membrane Selection: The choice of membrane affects proton conductivity, impacting the overall efficiency.
"A careful design of the fuel cell operating conditions can lead to improved efficiencies and greater energy output."
By understanding these chemical reactions and energy conversion mechanics, producers and researchers can better design and construct hydrogen fuel cells that meet specific requirements for various applications, from automotive to stationary power generation.
Essential Components of Hydrogen Fuel Cells
Understanding the essential components of hydrogen fuel cells is crucial for anyone interested in the development and operation of this sustainable energy technology. Each part serves a specific function, contributing to the overall performance and efficiency of the fuel cell. Awareness of these components not only aids in the construction process but also in troubleshooting issues that may arise.
Membrane Electrode Assembly
The Membrane Electrode Assembly (MEA) is at the heart of a hydrogen fuel cell. It consists of a proton exchange membrane sandwiched between an anode and a cathode side. The primary purpose of the MEA is to facilitate the electrochemical reaction that converts hydrogen and oxygen into electricity, water, and heat.
- Proton Exchange Membrane: This membrane allows protons to pass through while blocking electrons. This selective permeability is vital for generating electric current.
- Electrodes: The anode is where hydrogen gas is split into protons and electrons. This reaction generates electricity as electrons flow through an external circuit to the cathode. At the cathode, oxygen reacts with protons to form water.
The quality of the MEA significantly impacts the fuel cell's performance. Improvements in membrane materials can lead to greater efficiency and durability, making research in this area a high priority in fuel cell technology.
Bipolar Plates
Bipolar plates play a key role in fuel cell design by serving multiple functions. They separate individual cells within a stack and facilitate the flow of gases, while also conducting electrical current.
- Gas Distribution: Bipolar plates have channels that evenly distribute hydrogen and oxygen gases over the electrodes. Proper gas management is crucial for maintaining performance levels.
- Conductivity: Made from materials such as graphite or metal, bipolar plates must exhibit high electrical conductivity to minimize resistive losses.
- Cooling: They help manage heat generated during the electrochemical reactions. Effective thermal management ensures a stable operating temperature, which is essential for efficiency and longevity.
Adequate attention to the design and materials used for bipolar plates is necessary to prevent issues like corrosion that can arise over time.
End Plates and Gaskets
End plates and gaskets ensure the structural integrity and sealing of the fuel cell stack. These components are not just about support; they are integral to the overall function of the fuel cell.
- End Plates: They provide mechanical stability, joining different layers of the stack together while maintaining alignment. This is essential for operational reliability.
- Gaskets: These components are critical for sealing the assembly. They prevent gas leaks and help maintain the internal pressure needed for optimal performance. The choice of gasket material is important since they must withstand high temperatures and corrosive environments.
Overall, effective end plates and gaskets contribute to the safety and efficiency of the hydrogen fuel cell.
"The components of hydrogen fuel cells are deeply interconnected. A failure in one element can lead to a significant drop in overall performance."
Materials for Construction
The selection of materials for constructing hydrogen fuel cells greatly affects their performance, durability, and overall efficiency. In this section, we focus on the vital components and their significance in the fuel cell design. Choosing the right materials is critical for optimizing reaction rates and ensuring safety.
Types of Electrolytes
Electrolytes play a central role in fuel cells, facilitating the movement of ions while preventing electron transfer. The choice of electrolyte influences the operational temperature and the efficiency of the fuel cell. Common types include:
- Proton Exchange Membranes (PEM): These are widely used in hydrogen fuel cells due to their high ionic conductivity at low temperatures. They require hydration to function properly, making them suitable for automotive applications.
- Alkaline Electrolytes: These materials operate in basic conditions and often involve cheaper catalysts. They have historically been used in less demanding applications but are gaining attention in newer designs.
- Solid Oxide Electrolytes: These are suitable for high-temperature fuel cells and are known for their robustness. Their operational range allows them to use alternative fuels, broadening their use cases.
When selecting electrolytes, consider factors like conductivity, stability, and compatibility with other materials in the cell. Multiple options offer different advantages, allowing developers to tailor designs for specific applications.
Catalysts: Platinum and Alternatives
Catalysts are essential to facilitate the reaction at the anode and cathode in a hydrogen fuel cell. Platinum is the most efficient catalyst, known for its excellent performance in promoting hydrogen oxidation and oxygen reduction. However, its high cost and limited availability pose challenges for widespread adoption.
Alternatives to platinum include:
- Palladium: Has catalytic properties similar to platinum and is slightly more affordable.
- Nickel: Often used in alkaline fuel cells, nickel offers decent catalytic activity for hydrogen reactions at a lower cost.
- Carbon-based catalysts: These include various composite materials that can offer reduced costs and increased availability while maintaining reasonable performance levels.
Research continues into developing non-precious metal catalysts that could outperform or equal platinum. This would significantly reduce the cost of hydrogen fuel cells and enhance their commercial viability.
Structural Materials
The structural integrity of a hydrogen fuel cell affects its longevity and efficiency. Choosing appropriate materials ensures the stack can withstand operational stresses while retaining gas permeability.
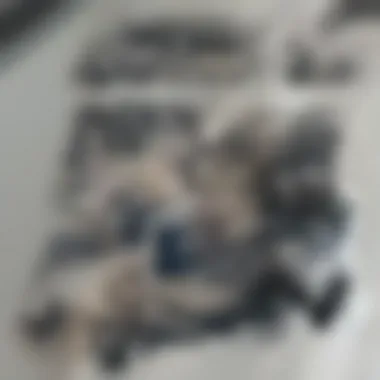
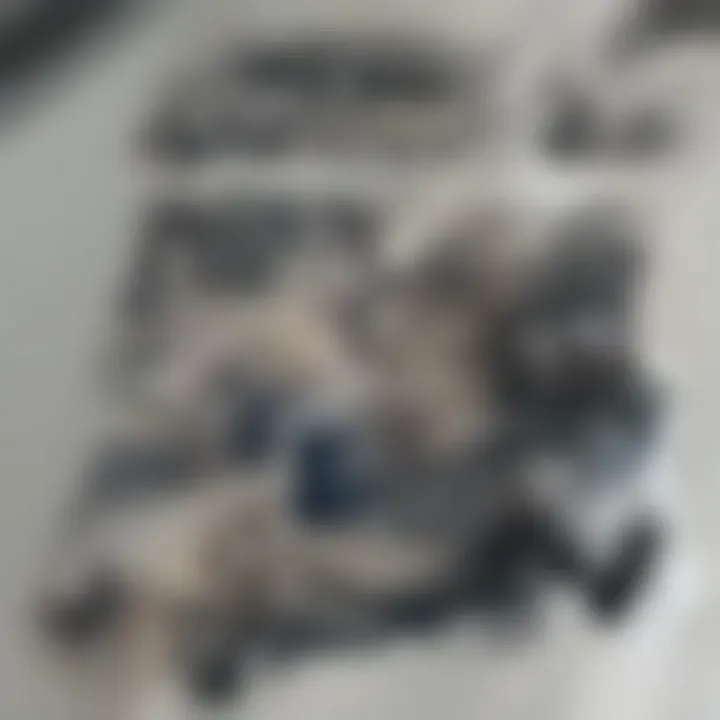
Key structural materials include:
- Graphite or Carbon Composites: Commonly used in bipolar plates because they provide good mechanical properties while allowing for efficient gas distribution and electrical conductivity.
- Stainless Steel: While less conductive, stainless steel is resistant to corrosion and can provide excellent durability in various environments.
- Plastics and Polymers: Often used for gaskets and seals, these materials prevent leaks and maintain the integrity of the fuel cell stack.
Overall, material selection influences not only performance but also the manufacturing cost and the sustainability of hydrogen fuel cell systems. Prioritizing durability, efficiency, and cost-effectiveness is necessary for the successful deployment of these technologies.
"The efficiency of the entire system can be limited by a suboptimal choice of materials. It's essential to consider the interplay between each component in the fuel cell stack."
Step-by-Step Construction Process
The construction process of a hydrogen fuel cell is a crucial step that determines its efficiency and reliability. This phase combines various skills and knowledge areas, from engineering design to intricate assembly techniques. Here, we will outline the step-by-step construction process including designing the fuel cell stack, assembling components, and ensuring testing and quality assurance.
Designing the Fuel Cell Stack
Designing the fuel cell stack is the first concrete step in the construction process. This stack will ultimately house the essential components that enable the chemical reaction between hydrogen and oxygen. The design must consider the number of cells, dimensions, and the configuration (series or parallel). Each cell’s individual characteristics impact the overall power output and efficiency.
Factors such as the expected operational environment and energy requirements must be evaluated. For instance, high power demands need stacks designed for rapid hydrogen flow and optimized surface area for reactions. Furthermore, the integration of a thermal management system is essential to regulate the temperature during operation, as excessive heat can degrade performance.
In addition to performance considerations, materials selection plays an integral role during design. Components within the stack should be durable and resistant to corrosive effects from the fuel cell operation. For example, the choice of membrane and bipolar plates directly influences the longevity and maintenance frequency of the fuel cell.
Assembling Components
Once the design phase is complete, the assembly of components begins. This process requires precision and patience. Each component must fit correctly to avoid leaks and ensure optimal flow of reactants. The critical components include the membrane electrode assembly, bipolar plates, and the end plates.
During assembly, it is essential to follow the design specifications closely. Start by carefully stacking the membrane electrode assemblies and sealing them with gaskets. This is where attention to detail becomes paramount.
- Important Tips During Assembly:
- Ensure that all surfaces are clean and free from contaminants.
- Use appropriate adhesives and seals to prevent hydrogen leakage.
- Properly align each component according to the pre-determined design to ensure maximum efficiency.
Working systematically to assemble these components will help maintain organization and coherence within the stack. Each step in the assembly can significantly impact the overall success of the fuel cell’s performance.
Testing and Quality Assurance
After assembly, the hydrogen fuel cell stack goes through rigorous testing. This stage is vital for identifying any potential flaws and verifying that the cell meets performance specifications. Testing can involve several phases, including functionality checks and stress testing.
Quality assurance measures should be in place to evaluate the integrity of the assembly. This includes checking the assembly for leaks, measuring performance metrics, and analyzing any deviations in expected outcomes.
- Testing Procedures May Include:
- Pressure Testing: Determines the resilience of the stack under operating conditions.
- Electrical Testing: Confirms that the cell produces the desired voltage and current output under various loads.
- Temperature Testing: Assesses how well the fuel cell can deal with operational thermal stresses.
Ultimately, proper testing guarantees that the hydrogen fuel cell will operate efficiently when deployed. The end result of a meticulous construction process is a functional and reliable energy source that holds the potential to contribute to the sustainable energy landscape.
Safety Considerations
Safety is of paramount importance when working with hydrogen fuel cells. Hydrogen is a flammable gas that can pose serious risks if not handled properly. Understanding safety considerations helps prevent accidents and ensures a safe working environment. Moreover, adhering to proper safety protocols can enhance the longevity and efficiency of the hydrogen fuel cell itself. Key aspects of safety in this context include hydrogen handling procedures and electrical safety measures.
Hydrogen Handling Procedures
Hydrogen handling is critical for preventing leaks and explosions. The following guidelines can be applied to ensure safe handling:
- Storage: Store hydrogen in well-ventilated areas away from ignition sources. Use appropriate cylinder storage racks to prevent tipping.
- Leak Detection: Implement hydrogen detectors for early leak detection. Regularly inspect seals and valves in storage tanks and fuel cells to identify potential leaks.
- Personal Protective Equipment (PPE): Wear safety goggles, gloves, and protective clothing when working with hydrogen. This reduces the risk of injury in case of an accident.
- Training and Protocols: Ensure that all personnel involved in handling hydrogen undergo proper training. Establish standard operating procedures to minimize risk, including emergency response plans.
"When hydrogen is handled without care, the consequences can be catastrophic. Prevention is always better than remediation."
By focusing on the specific procedures for safely managing hydrogen, individuals can contribute to a more secure working environment.
Electrical Safety Measures
Electrical safety is another essential consideration in the operation of hydrogen fuel cells. Improper electrical management can lead to shocks or fires. Here are some measures to enhance electrical safety:
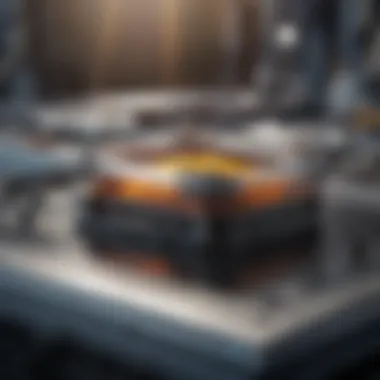
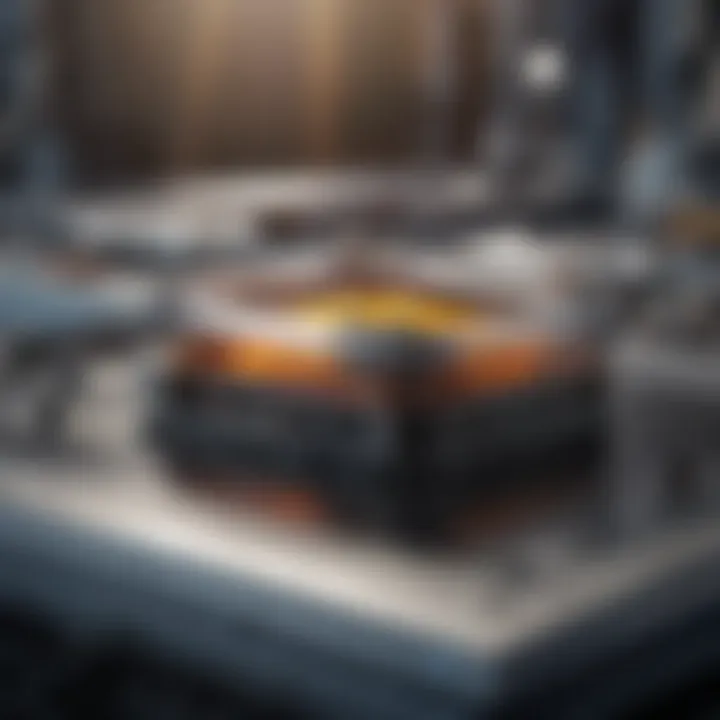
- Grounding: Always ground electrical systems to prevent voltage buildup. This reduces the risk of electric shock and protects sensitive equipment.
- Circuit Protection: Install fuses and circuit breakers to prevent overheating and electrical fires. Both help by cutting off power during an electrical fault.
- Regular Inspections: Schedule regular inspections of all electrical components. Look for wear and tear, especially in wiring and connectors, to ensure everything is functioning as intended.
- Use Non-Sparking Tools: When working around hydrogen, use tools designed to minimize the risk of sparks. Non-sparking tools will further reduce the risk of ignition in case of a hydrogen leak.
These safety measures are essential for anyone working with hydrogen fuel cells. Proper safety protocols ensure greater confidence in the technology and minimize potential hazards.
Optimization of Performance
Optimizing the performance of hydrogen fuel cells is crucial in enhancing their efficiency and reliability. As these cells operate, various factors influence their overall output, including electrical efficiency, durability, and strength. By addressing these elements, researchers and engineers can ensure that fuel cells function at their best while minimizing potential failures. The following sections delve into specific areas crucial for optimization in hydrogen fuel cell creation.
Operating Conditions
Operating conditions refer to the environment in which the fuel cell functions. These conditions significantly impact performance, usability, and lifecycle. The key factors to consider in optimizing operating conditions include:
- Temperature: Operating at optimal temperatures enhances reaction rates. Generally, a range between 60°C to 80°C offers improved efficiency, but exceeding limits can degrade materials.
- Pressure: Pressure adjustments can affect hydrogen and oxygen availability. Higher pressures usually lead to higher output, yet they increase mechanical stress on components.
- Humidity: Maintaining proper humidity levels is vital to electrolyte functioning. Too little humidity can cause drying, affecting proton conductivity. Conversely, excessive moisture leads to flooding, which hampers gas flow.
In summary, controlling these conditions is essential for the maximization of hydrogen fuel cell performance.
Troubleshooting Common Issues
Despite careful construction and optimization, hydrogen fuel cells may experience common problems. Identifying and resolving these issues promptly is crucial for maintaining performance and safety. Some prevalent troubles include:
- Decreased Efficiency: If the efficiency of the fuel cell drops, it may suggest issues such as blockage in the membrane or degradation of the catalyst. Routine checks to verify the condition of these components help address the problem swiftly.
- Leakage: Hydrogen leaks pose a severe safety hazard. Regular inspections for fittings and seals can prevent leaks before they escalate into emergencies.
- Voltage Fluctuations: Unstable voltage outputs can arise from incorrect operating conditions or component failures. Analyzing the electrical connections and overall system performance can help remedy this issue.
*Regular maintenance and monitoring are fundamental to avoiding prolonged downtimes or costly repairs.
Through understanding and addressing these operating conditions and troubleshooting measures, one can optimize hydrogen fuel cell performance effectively, contributing to the advancement of this sustainable energy technology.
Future Directions in Hydrogen Fuel Cell Technology
The exploration of future directions in hydrogen fuel cell technology is essential for advancing sustainable energy solutions. The importance of this topic is rooted in its potential to reduce reliance on fossil fuels and improve energy efficiency. There are several specific elements to consider when looking ahead in the field of hydrogen fuel cells.
Advancements in materials science, including polymer electrolytes and innovative catalysts, can enhance fuel cell performance. Research in these areas seeks to lower costs while maximizing durability and efficiency. Moreover, scaling up production methods is critical for making fuel cells more widely accessible.
Emerging Research Areas
Emerging research areas focus on both fundamental and applied aspects of hydrogen fuel cells. Key areas include:
- Alternative Catalysts: Research is ongoing in developing non-platinum catalysts, which can significantly lower production costs. Metal oxides and transition metal nitrides are receiving attention.
- Enhanced Membrane Technology: New ion exchange membranes can improve proton conductivity and thermal stability. This leads to higher cell performance under various operating conditions.
- Hydrogen Production Methods: Sustainable hydrogen production is crucial. Electrolysis methods, especially those using renewable energy sources, are an active research area.
These areas represent promising developments. They could overcome current limitations and enhance the commercial viability of hydrogen fuel cells.
Commercialization and Market Trends
Commercialization represents a significant milestone for hydrogen fuel cells. The market has begun to evolve steadily, driven by increased demand for clean energy solutions. Some of the significant trends include:
- Automotive Applications: Major automotive manufacturers like Toyota and Honda are investing in hydrogen fuel cell vehicles. This trend reflects growing consumer interest in zero-emission transportation.
- Utility-Scale Projects: Hydrogen is finding its place in large-scale energy storage and delivery systems. These projects can integrate renewable energy sources, providing a balanced energy mix.
- Policy Support: Government incentives and subsidies for hydrogen technologies are on the rise. This can accelerate research and increase market penetration.
As the market continues to develop, collaboration between academia, industry, and government will be crucial. This approach can drive innovation and facilitate the transition towards a hydrogen-based economy.
"In order to fully realize the potential of hydrogen fuel cells, we must focus on both technology advancement and market strategies that align with global sustainability goals."
In summary, the future directions in hydrogen fuel cell technology highlight the need for ongoing research and market adaptations. As these developments unfold, they will play a critical role in shaping a more sustainable energy landscape.
Culmination
In this article, we explored the construction of hydrogen fuel cells, emphasizing the intricate details that contribute to their functionality. Hydrogen fuel cells symbolize a shift towards sustainable energy solutions. The construction process involves understanding various components, materials, and operational mechanics. Each part plays a critical role in ensuring efficiency and reliability.
Summarizing Key Takeaways
- Understanding the core principles of fuel cell operation is essential. The breakdown of hydrogen and oxygen leading to energy creation opens up new avenues for power generation.
- The selection of materials, especially electrolytes and catalysts, significantly impacts the overall performance of the fuel cell. Platinum remains a leader, though alternatives are emerging.
- Safety considerations cannot be overlooked. Hydrogen is flammable and needs proper handling protocols.
- Optimization of operating conditions can drastically improve efficiency. Careful monitoring during use, as well as regular maintenance, ensures long-term viability.
Implications for Sustainability
Hydrogen fuel cells present a promising alternative to fossil fuels, which have long been a staple of energy consumption. They offer a clean energy source. The only byproduct is water, reducing environmental pollution significantly. This shift aligns with global sustainability goals.
- Clean Energy Transition: Adopting hydrogen technologies supports broader efforts to minimize carbon footprints.
- Further Research: Continued advancements in fuel cell technologies can lead to lower costs and increased efficiency, making them more accessible.
- Economic Impact: A growing hydrogen sector could stimulate job creation and investment, benefiting economies worldwide.
In summary, hydrogen fuel cells not only represent a technological evolution but also a pathway to sustainable energy practices. Understanding their construction and operation is vital for future energy systems.