Hydrogen to Electricity Efficiency: A Comprehensive Analysis
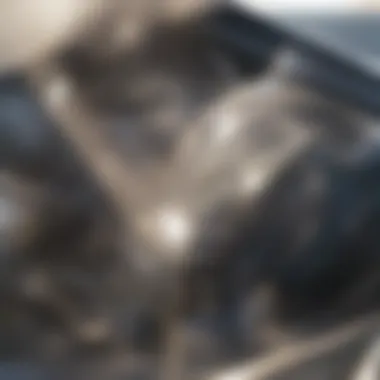
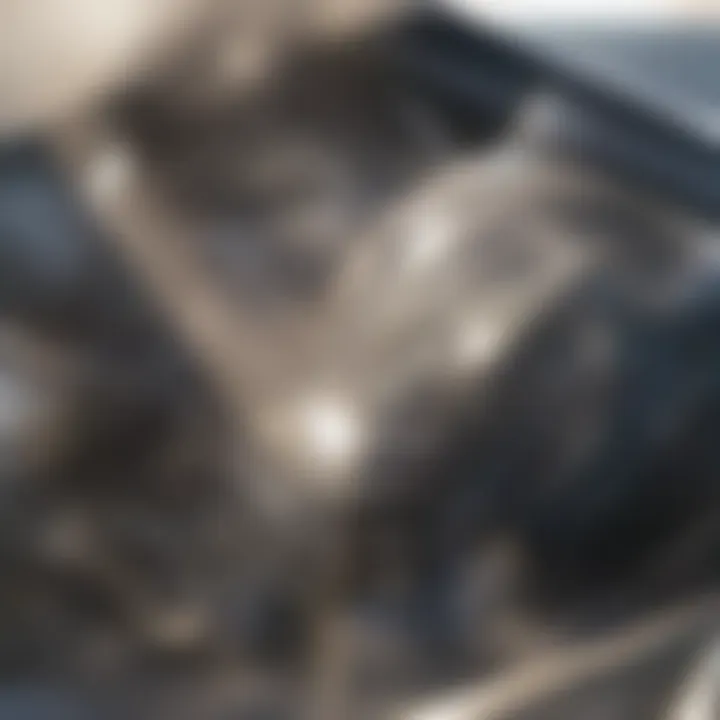
Intro
The transition to renewable energy systems is becoming more apparent. Hydrogen stands out as a pivotal element in this shift. The conversion of hydrogen to electricity offers an intriguing area of research, especially considering current concerns over energy storage and sustainability.
Understanding the efficiency of this conversion process is essential. As energy demand rises, the need for effective methods to generate electricity from hydrogen cannot be overstated. This article intends to detail the different techniques involved in this conversion and assess their overall performance.
Research Overview
Summary of Key Findings
In recent years, various methods for converting hydrogen to electricity have gained attention. Key findings highlight that:
- Fuel cells are one of the most efficient technologies available, converting chemical energy directly to electrical energy.
- Efficiency rates vary significantly by technology, with some systems achieving over 60% efficiency under optimal conditions.
- The full utilization of hydrogen's potential is contingent upon addressing storage and distribution challenges.
These insights reveal the potential of hydrogen as a clean energy source, especially when complemented by renewable resources.
Significance of Research
The dynamics of energy consumption are ever-changing. As countries focus on reducing carbon footprints and embracing renewable resources, hydrogen becomes increasingly significant. Research into hydrogen-to-electricity conversion not only fosters innovation but also supports policy-making in the energy sector. By understanding how hydrogen can effectively generate electricity, stakeholders can make informed decisions that contribute to sustainable futures.
Methodology
Research Design
The research documented in this article employs a qualitative framework. This approach allows for an in-depth examination of existing technologies and their efficiencies. By reviewing case studies and existing literature, a comprehensive understanding emerges.
Data Collection Methods
Data for this research is gathered through multiple methods:
- Literature Review: Analyzing previous studies on hydrogen conversion.
- Case Studies: Learning from real-world applications and their efficiencies.
- Expert Interviews: Gathering insights from professionals working in the field.
These methods ensure a robust analysis, facilitating the synthesis of detailed findings.
"The efficiency of converting hydrogen to electricity is not merely an academic topic; it is central to the future of sustainable energy strategies."
This examination into the efficiency of hydrogen-to-electricity conversion paves the way for future research and discussions. Understanding the conversion process, its methodologies, and implications is crucial for advancing energy systems.
Preamble to Hydrogen as an Energy Carrier
Hydrogen is emerging as a vital component in the quest for sustainable energy solutions. As we strive to minimize carbon emissions and diversify energy sources, hydrogen offers promising advantages as an energy carrier. This section will explore the significance of hydrogen in the current energy landscape and its potential benefits.
Hydrogen energy serves as an effective medium for storing and transporting energy. It can generate electricity through various methods, such as fuel cells and combustion. This versatility positions hydrogen as a complementary resource to intermittent renewable sources, like solar and wind. Hydrogen's abundance and the potential for clean production methods further enhance its appeal.
There are critical considerations associated with hydrogen utilization. Safety, efficiency, and infrastructure readiness are pivotal in its widespread adoption. For example, the energy loss during conversion processes can limit overall efficiency. Addressing these concerns will be essential for realizing hydrogen's full potential in the global energy mix.
Defining Hydrogen Energy
Hydrogen energy refers to the use of hydrogen as a fuel or energy source to produce power. It exists in nature predominantly in compound form, such as water. The process of hydrogen production involves separating it from these compounds. Hydrogen can be generated through methods like electrolysis, where electricity splits water molecules, or from fossil fuels through processes such as steam methane reforming.
When utilized in fuel cells, hydrogen combines with oxygen to produce electricity, water, and heat. This clean method of generation results in minimal emissions, making hydrogen energy appealing for reducing greenhouse gas output. Nevertheless, understanding the various production methods is crucial as they dictate the emissions associated with hydrogen use.
Historical Context of Hydrogen Usage
The journey of hydrogen as an energy source spans several decades. Its significance was first recognized in the early 1800s when scientists began isolating hydrogen. The development of internal combustion engines in the 19th century sparked interest in hydrogen as a fuel. However, the rise of petroleum-based fuels led to diminished focus on hydrogen technologies.
In recent years, the notion of hydrogen as an alternative fuel has resurfaced, driven by advances in technology and growing environmental concerns. Hydrogen fuel cells gained traction in the transportation sector, as seen with vehicles like the Toyota Mirai.
"Hydrogen is a promising energy carrier and can bridge the gap towards a sustainable energy future."
As countries pursue energy independence and sustainability, historical lessons can inform current efforts. The advancements in hydrogen production and utilization underscore its long-forgotten potential.
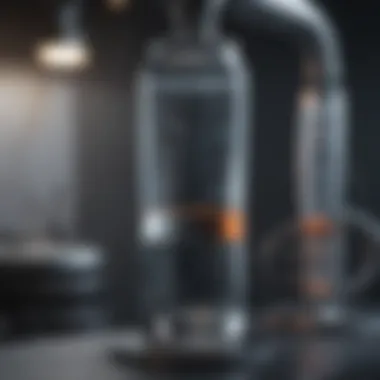
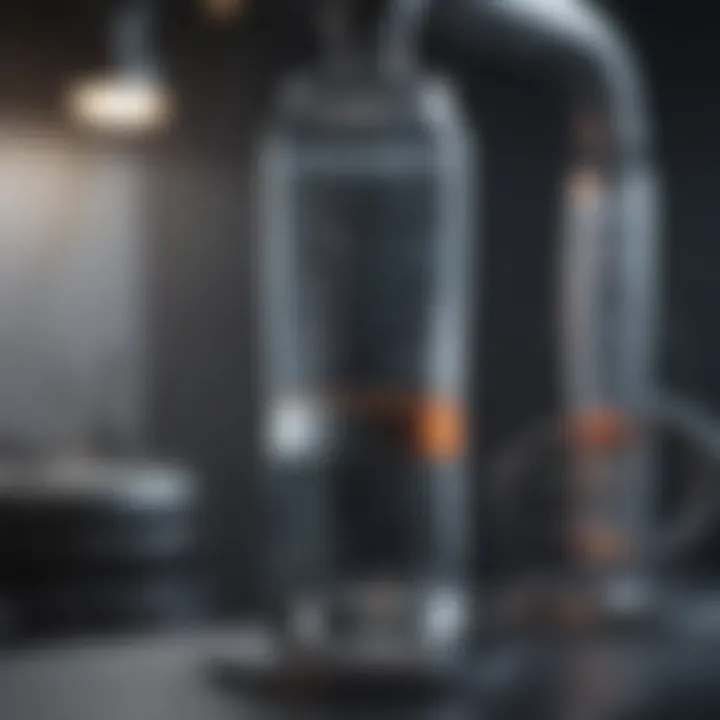
In summary, hydrogen presents numerous advantages in energy storage and transmission. While challenges persist, the historical context highlights a cycle of interest and innovation that could redefine its role in modern energy systems.
The Chemistry Behind Hydrogen Energy Conversion
Understanding the chemistry of hydrogen energy conversion is crucial for optimizing the efficiency of processes that transform hydrogen into electricity. Hydrogen is unique due to its simplicity and reactivity; it has only one electron and proton. This simplicity lends itself to various reactions that can be harnessed for energy production. By studying the chemical properties and reactions involved, researchers can gain insights into system performance and limitations. The efficiency metrics in hydrogen conversion largely rely on the chemistry at play. Consequently, advancements in this area can lead to innovations in energy technology, ensuring a sustainable energy future.
Chemical Properties of Hydrogen
Hydrogen exists as a diatomic gas, represented chemically as . As the lightest element, it plays a significant role in various chemical reactions. It's non-toxic, and when combusted, hydrogen produces only water vapor as a byproduct, making it an environmentally friendly option. Moreover, hydrogen has a high specific energy content, approximately 120 MJ/kg, which makes it an efficient fuel source when compared with traditional fossil fuels. This high energy density allows for its potential use in diverse applications ranging from powering vehicles to generating electricity. Understanding these chemical properties is essential when developing systems that utilize hydrogen for energy.
Electrochemical Reactions in Fuel Cells
Fuel cells represent a critical technology for converting hydrogen into electricity through electrochemical reactions. In a fuel cell, hydrogen molecules undergo oxidation at the anode, releasing electrons and hydrogen ions. This reaction generates a flow of electric current as the electrons move through an external circuit. Simultaneously, hydrogen ions migrate through a membrane to the cathode, where they react with oxygen to form water and heat as byproducts.
The efficiency of this process is influenced by several factors, including:
- Material Quality: The type of membrane and electrodes used can impact the reaction rates.
- Temperature and Pressure: These conditions must be optimized for maximum efficiency.
- Reaction Kinetics: Faster reactions typically lead to higher overall system efficiency.
Understanding these electrochemical principles is essential for advancing fuel cell technology, aiming to overcome current limitations and enhance performance.
Thermochemical Processes
Thermochemical processes offer an alternative means of converting hydrogen into electricity. These processes rely on the heat generated during chemical reactions to drive endothermic reactions, which effectively produce hydrogen or electricity. Two common thermochemical cycles used for hydrogen production are the sulfur-iodine cycle and the copper-chlorine cycle.
In the sulfur-iodine cycle, concentrated solar heat decomposes sulfuric acid to release hydrogen, while in the copper-chlorine cycle, heat from nuclear reactors drives the reactions. The efficiency of thermochemical processes can be affected by:
- Heat Source Characteristics: The temperature and efficiency of the heat source directly influence the conversion process.
- Reaction Management: Controlling reaction conditions can enhance yields and efficiency.
- Material Stability: The durability of materials at high temperatures can affect the long-term viability of the process.
Overall, understanding the thermochemical methods of hydrogen energy conversion is important for developing alternative pathways that can complement existing technologies.
Key Insight: The chemistry behind hydrogen energy conversion encompasses both electrochemical and thermochemical methods, each with distinct advantages and challenges, significantly impacting the efficiency of hydrogen as an energy carrier.
Methods for Converting Hydrogen to Electricity
The conversion of hydrogen to electricity is a critical topic in today's energy landscape. As the world aims to transition towards sustainable energy solutions, understanding the various methods and technologies for this conversion is essential. These methods not only influence the efficiency of hydrogen utilization but also determine its viability as a key player in future energy systems.
Different means of converting hydrogen into electricity include fuel cells and combustion engines, each with its own technological framework and operational characteristics. Exploring these methods allows researchers, professionals, and policymakers to assess the potential and limitations of hydrogen as an energy carrier in various applications.
Fuel Cell Technology
Fuel cell technology represents one of the most promising methods for converting hydrogen into usable electricity. Fuel cells operate by utilizing hydrogen, which combines with oxygen through electrochemical reactions to produce electric power, water, and heat. They offer high efficiency and low emissions, making them an important technology in the move towards clean energy solutions.
Proton Exchange Membrane Fuel Cells
Proton Exchange Membrane Fuel Cells (PEMFCs) are notable for their lightweight and compact design. They operate at relatively low temperatures, around 80 degrees Celsius, making them ideal for portable applications and vehicles. The key characteristic of PEMFCs is their ability to provide quick response times and high power density.
This technology is a beneficial choice for applications where efficiency and compactness are essential, such as in automotive and portable power applications. A unique feature of PEMFCs is their membrane that conducts protons while being impermeable to gases, thus enhancing safety and performance. However, the disadvantages include sensitivity to fuel impurities and relatively high costs of materials like platinum used in the catalyst layer.
Solid Oxide Fuel Cells
Solid Oxide Fuel Cells (SOFCs) operate at much higher temperatures, typically between 600 and 1000 degrees Celsius. This high operating temperature allows for the utilization of a variety of fuels, including natural gas and biogas, alongside hydrogen. The key characteristic of SOFCs is their high efficiency and ability to achieve a greater overall energy output.
SOFCs are considered a popular choice for stationary power generation where efficiency is crucial. They can achieve efficiencies greater than 60%, making them an attractive option for large scale energy applications. A unique feature of SOFCs is their ability to operate in both fuel cell and electrolysis modes, thus offering flexibility in energy scenarios. However, their disadvantages include longer startup times and the need for thermal management due to high operating temperatures.
Molten Carbonate Fuel Cells
Molten Carbonate Fuel Cells (MCFCs) are another type of high-temperature fuel cell, operating around 600 degrees Celsius. They utilize a molten carbonate salt as the electrolyte, enabling good ionic conductivity. The key characteristic of MCFCs is their capability to use various fuels, which makes them highly versatile.
This type of fuel cell is a beneficial option for large-scale, stationary energy production. They offer high overall efficiency and can utilize waste CO2 from industrial processes. A unique feature of MCFCs is their ability to operate at high current densities. However, they have disadvantages such as corrosion challenges and limited application in portable scenarios due to their larger physical size.
Hydrogen Combustion Engines
In addition to fuel cells, hydrogen combustion engines represent another approach to convert hydrogen into electricity. These engines function similarly to conventional internal combustion engines but burn hydrogen instead of fossil fuels. This method can achieve substantial efficiencies when optimized for hydrogen use. While hydrogen combustion engines can be easier to implement using existing engine technologies, their overall efficiency may be lower compared to fuel cells, primarily due to thermal losses during combustion.
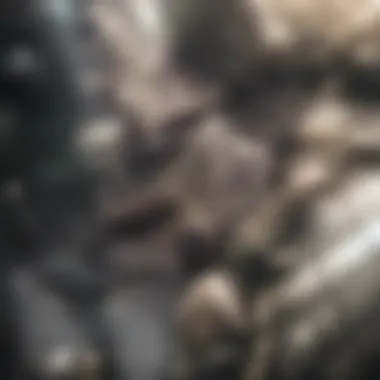
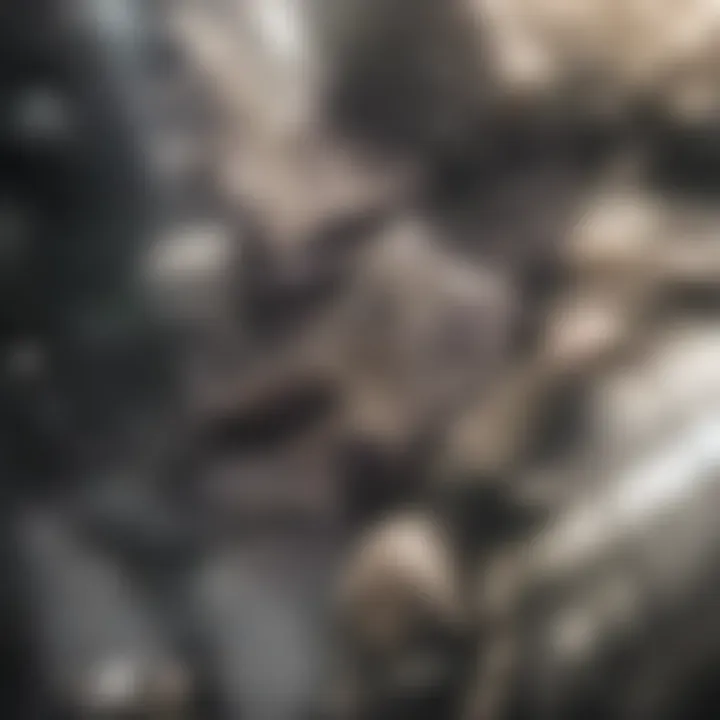
Each of these methods presents unique advantages and challenges. The choice of technology will depend on application requirements, scalability, and cost considerations. Understanding these conversion methods is essential for advancing the role of hydrogen in sustainable energy solutions.
Efficiency Metrics in Hydrogen Conversion
The efficiency of converting hydrogen to electricity is a critical aspect in realizing hydrogen's potential as a clean energy carrier. Efficient conversion not only determines the viability of hydrogen technologies but also influences their economic feasibility. In this context, understanding the metrics of efficiency can guide the optimization of hydrogen systems, enhancing both environmental and economic performance. Key factors include energy yield, conversion technologies employed, and external conditions affecting performance.
Factors Influencing Efficiency
Temperature and Pressure Conditions
Temperature and pressure are vital in optimizing the efficiency of hydrogen conversion processes. Higher temperatures often enhance reaction rates in electrochemical and thermochemical systems; however, they may also increase losses due to heat dissipation. Pressure plays a dual role, as higher pressures can raise efficiency in certain reactions but may require more energy to maintain.
- A key characteristic of optimal temperature and pressure settings is their ability to maximize reaction kinetics while minimizing energy losses.
- The unique feature here is the balance that must be struck to achieve maximum efficiency, as extreme conditions may lead to diminishing returns. For instance, while elevated pressures can enhance fuel cell performance, they also add complexity and cost to system designs.
Material Selection
Material selection directly influences the overall efficiency of hydrogen conversion systems. Materials used for electrodes, membranes, and structural components need to exhibit favorable electrochemical properties alongside durability under operational conditions.
- A prominent characteristic here is the conductivity of materials; higher conductivity can lead to lower resistive losses during energy conversion.
- The unique feature in material selection is the trade-off between performance and cost. For example, platinum is highly effective as an electrode material due to its catalytic properties, yet it is expensive, making its widespread use less feasible.
System Design
The design of hydrogen conversion systems plays a crucial role in determining efficiency. Considerations such as the layout of components, integration of systems, and thermal management strategies contribute to overall performance.
- A fundamental characteristic of efficient system design is its ability to minimize energy losses at various stages of conversion.
- The unique feature of innovative designs is their adaptability to different scales and contexts. For example, modular designs can enhance efficiency in distributed energy systems but may encounter limitations in central large-scale applications.
Measuring Efficiency: Input vs. Output Energy
Measuring efficiency in hydrogen conversion involves comparing the input energy required for processes against the output energy generated. This metric is essential for evaluating the performance of various conversion technologies, including fuel cells and combustion systems.
Efficiency can be expressed through the following formula:
Understanding input and output energy values allows researchers to identify areas for improvement within hydrogen conversion systems, providing a clear pathway for enhancing performance as technologies evolve.
Challenges in Hydrogen to Electricity Conversion
The conversion of hydrogen to electricity holds significant promise, yet various challenges impede its widespread adoption. Understanding these challenges is critical for researchers, policymakers, and industry professionals. Addressing these issues could enhance the efficiency and viability of hydrogen as a clean energy carrier.
Cost of Production and Infrastructure
The cost of producing hydrogen is one of the primary challenges. Currently, methods such as steam methane reforming and electrolysis are common, yet they have limitations. Steam methane reforming relies on natural gas, which can be costly and environmentally taxing. Electrolysis, while cleaner, requires substantial electrical energy, making it less cost-effective, especially when using renewable sources.
Infrastructure also poses a challenge. Transporting and storing hydrogen necessitates specialized pipelines and tanks, which may not be economically feasible in many regions. Existing energy infrastructures are typically designed for fossil fuels, further complicating the transition to hydrogen systems.
Efforts to develop economic strategies in both production and infrastructure development are essential. The consolidation of production facilities, increased efficiency in renewable energy integration, and advancements in storage technology may lower costs in the long run.
Storage and Transportation Issues
Hydrogen storage presents unique challenges. It can have a low volumetric energy density, necessitating high-pressure storage or liquefaction. Both methods require advanced technologies that could increase costs and complexity. Moreover, transporting hydrogen safely across long distances is not straightforward, as hydrogen is prone to leakage and embrittlement of materials.
Innovations in storage solutions are vital. Development of chemical hydrogen storage, where hydrogen is bound to other materials, can offer safer options compared to traditional high-pressure tanks. Similarly, advances in transport methods, like using modified natural gas pipelines, could provide more efficient means for moving hydrogen to end-users.
These challenges make it imperative for innovators to rethink hydrogen storage and transportation solutions to ensure that hydrogen can be integrated effectively into energy systems.
"Addressing cost and infrastructure problems is fundamental for realizing hydrogen as a prominent player in future energy landscapes."
The ability to convert hydrogen to electricity efficiently may hinge on breakthroughs in production economics and the establishment of robust infrastructures. This holistic perspective is necessary to harness the potential that hydrogen offers.
Comparative Efficiency with Other Energy Sources
Understanding the efficiency of hydrogen in comparison to other energy sources is crucial in assessing its viability as a sustainable energy carrier. This section focuses on how hydrogen stacks up against traditional and emerging technologies in energy production, including batteries and renewables. By examining the comparative efficiencies, we can identify potential advantages and existing limitations of hydrogen.
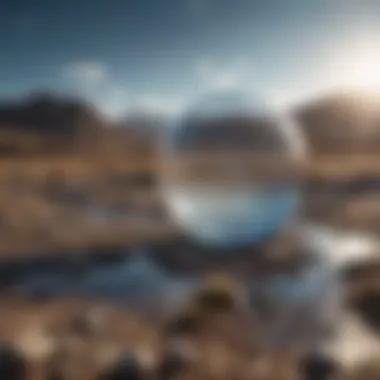
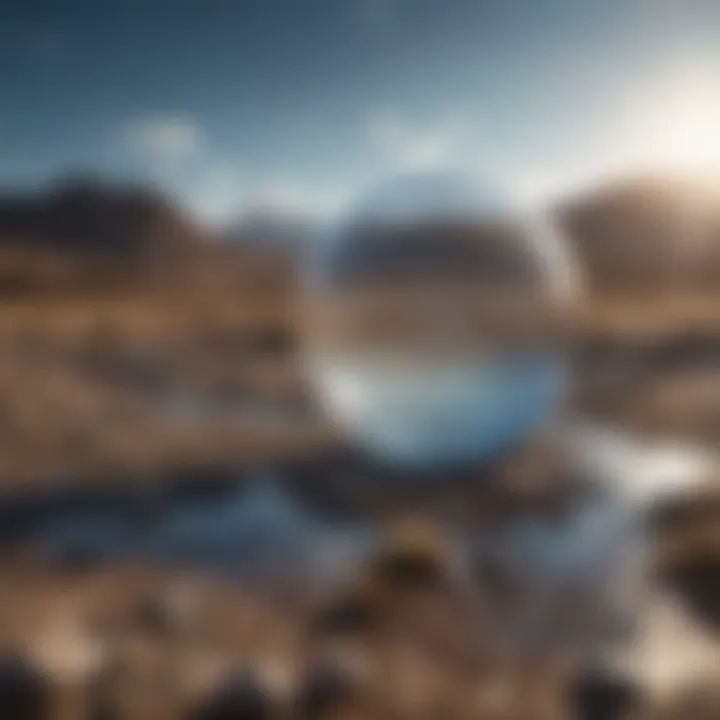
The use of hydrogen as an energy source brings some unique benefits. First, it has a high energy density, which means that a smaller volume can produce more energy. This characteristic makes it suitable for various applications, including transportation and long-term energy storage. Hydrogen can be produced from various materials, including water, biomass, and fossil fuels, creating a diverse range of feedstocks for energy generation.
Considerations include:
- Energy conversion efficiency
- Impact on greenhouse gas emissions
- Overall system costs
Examining these aspects will help determine where hydrogen may fit into the broader energy landscape, especially in integrating various energy solutions for more sustainable infrastructures.
Hydrogen versus Batteries
When comparing hydrogen with batteries, it is essential to look at various metrics. Batteries, particularly lithium-ion batteries, excel in applications that require quick response times and high efficiency for shorter durations. They are heavily relied upon in the electric vehicle sector and portable electronic devices.
However, batteries have inherent limitations, especially regarding energy density and discharge rates. As a general rule, batteries provide adequate short-term solutions where immediate energy demands are critical. In contrast, hydrogen can offer long-term storage capabilities and is particularly advantageous in large-scale applications, like grid energy storage, where renewable sources may not align with demand.
Key points for comparison:
- Energy density: Hydrogen has a greater energy density than batteries, making it more suitable for long durations.
- Refueling time: Hydrogen fuel cells can be refilled more quickly than battery charging.
- Lifecycle: The longevity of hydrogen systems may allow a broader range of applications without as frequent replacements as batteries.
Each energy source has its strengths and weaknesses, leading to a scenario where they can be complementary rather than one being superior to the other. While batteries dominate the market for specific uses, hydrogen shows promise in areas where efficiency and longer storage solutions are critical.
Role of Hydrogen in Renewable Energy Systems
As renewable energy sources like solar and wind power continue to grow, hydrogen's role becomes more significant. These energy systems often produce electricity when demand is low, leading to potential energy wastage. Hydrogen provides a solution by enabling excess renewable energy to be converted into hydrogen for later use.
In this regard, hydrogen acts as a storage medium that can balance supply and demand effectively. This capability helps mitigate the intermittency issues faced by renewables. When stored hydrogen is converted back to electricity, it can feed into the grid during peak demand periods.
Benefits of integrating hydrogen include:
- Energy storage: Hydrogen can store large amounts of energy over long periods, solving seasonal storage challenges.
- Grid stability: Hydrogen increases the flexibility of the energy grid by providing backup power during shortages.
- Decarbonization potential: Using hydrogen in industries and transport can significantly reduce carbon emissions, aligning with global sustainability goals.
In summary, hydrogen's integration into renewable energy systems offers a pathway toward a sustainable energy future. By blending hydrogen with other energy sources, we can enhance energy security and support a more resilient grid.
Future Directions in Hydrogen Energy Research
The research landscape surrounding hydrogen energy is rapidly evolving, with innovations that could significantly enhance its role in global energy systems. Future directions in hydrogen energy research are vital because they address critical challenges and opportunities for cleaner, more efficient energy solutions. The emphasis on hydrogen's potential within sustainable energy frameworks resonates with climate goals and energy transition strategies. By exploring developments in technology, production methods, and integration systems, researchers can unlock the true capabilities of hydrogen.
Innovative Technologies in Development
Innovative technologies are central to advancing hydrogen energy. There are several key areas where research is currently focused:
- Electrolysis Improvements: Enhanced alkaline and proton exchange membrane (PEM) electrolysis technologies aim to lower costs and increase efficiency. This can help produce green hydrogen from renewable sources such as wind and solar more effectively.
- Hydrogen Fuel Cells: Ongoing advancements in fuel cell technology are making them more efficient and cost-effective. Next-generation fuel cells are being developed to operate at higher temperatures and pressures, which can improve performance and durability.
- Hydrogen Storage Solutions: Effective storage solutions are critical for handling hydrogen safely and efficiently. Research is progressing into materials like metal hydrides and liquid organic hydrogen carriers (LOHCs), which offer potential answers to storage challenges.
- Biological Hydrogen Production: Exploration of biological methods using microorganisms to produce hydrogen could provide sustainable production avenues. This is a promising area, aiming to utilize waste and biomass to generate energy.
"The transition to hydrogen as a clean energy carrier depends heavily on continued technological advancements and economic scalability."
Scaling up Hydrogen Solutions
Scaling up hydrogen solutions presents both opportunities and challenges. Strategies to enhance the adoption of hydrogen technology in energy systems involve:
- Integration with Renewable Energy: Hydrogen can serve as an energy buffer for variable renewable sources. This capability means integrating hydrogen production with wind, solar, and tidal energy to stabilize the grid while ensuring energy availability.
- Infrastructure Development: Building an infrastructure for hydrogen distribution, storage, and refueling is crucial. Collaborative efforts between industries, governments, and researchers can facilitate the rollout of necessary infrastructure at scale.
- Policy Incentives: Policymakers must create favorable conditions through regulations and incentives for hydrogen development. This could include funding for research initiatives or grants for deploying hydrogen technologies in existing systems.
- Public Acceptance and Education: Public perception is essential for the hydrogen economy's growth. Informing stakeholders about the benefits and safety of hydrogen can encourage wider acceptance.
Through targeted efforts in these areas, the hydrogen sector can position itself as a cornerstone of future energy solutions. The potential for hydrogen energy to support decarbonization and sustainable development reinforces the need for ongoing research and commitment from all stakeholders.
End: The Path Forward for Hydrogen Energy
As we navigate the evolving landscape of energy production and consumption, particularly in the context of climate change, hydrogen energy emerges as a pivotal player. This article highlights the significance of examining hydrogen's role in generating electricity, especially as a complement to renewable energy sources. The future of hydrogen energy lies in its ability to integrate effectively into the existing energy framework.
Sustainability Implications
Hydrogen energy carries substantial sustainability implications. It operates on the principle of using abundant resources, often produced from water through electrolysis. By tapping into renewable sources like solar or wind energy for hydrogen production, we can substantially reduce greenhouse gas emissions. The cycle of producing, storing, and converting hydrogen into electricity can be optimized to yield lower carbon footprints.
However, challenges persist in making hydrogen a truly green option. The most common methods of hydrogen production, such as steam methane reforming, still rely on fossil fuels. Therefore, advancing technologies that enable cleaner production methods is crucial. This includes scaling up investments in electrolysis and exploring biohydrogen production. Additionally, public policies must adapt to incentivize cleaner hydrogen production and its applications in various sectors.
Integrating Hydrogen into the Energy Mix
Integrating hydrogen into the energy mix is essential for achieving a balanced and resilient energy system. As energy demands fluctuate, hydrogen can function as an energy storage solution. When renewable energy generation exceeds demand, excess electricity can convert into hydrogen. This stored hydrogen can later generate electricity during high-demand periods or when renewables are not producing energy.
- Role in Energy Security: Hydrogen contributes to energy security by diversifying energy resources. Countries can harness local renewable sources to produce hydrogen, reducing reliance on imported fuels.
- Interconnectivity with Other Technologies: The overlap of hydrogen technology with electric grids and other energy systems presents new opportunities. Hydrogen fuel cells can power transportation systems, while hydrogen blending in natural gas networks offers transitional pathways in existing infrastructures.
In summary, the path forward for hydrogen energy is marked by its potential to transform energy systems sustainably. As researchers and practitioners further study and develop hydrogen applications, the benefits will extend beyond simply generating electricity. The harmonious integration of hydrogen into our energy mix may well propel us towards a more sustainable and resilient future.