Instruments for Measuring Temperature: A Complete Guide
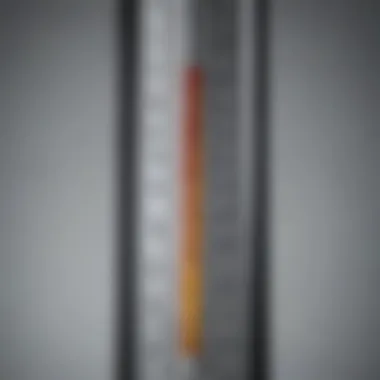
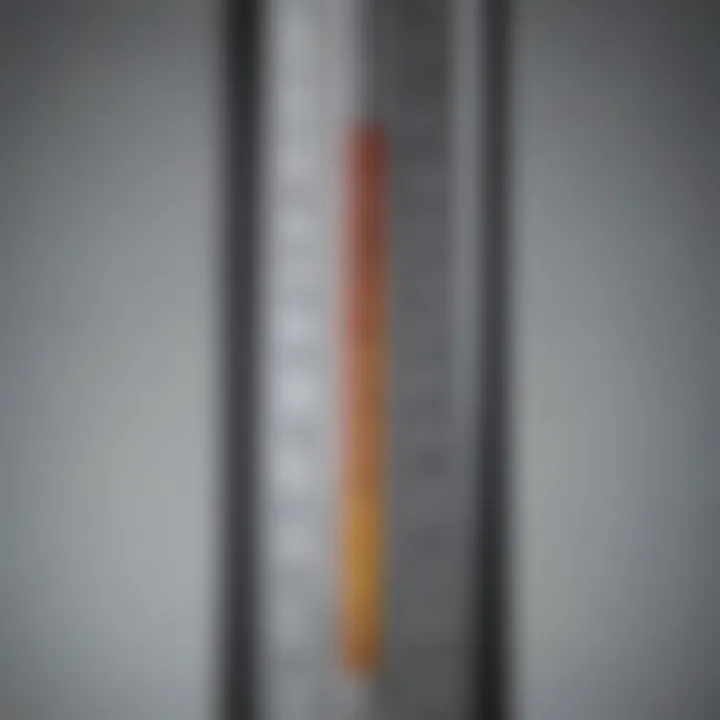
Intro
Temperature measurement is a fundamental aspect of both scientific investigation and industrial processes. As temperature plays a critical role in various applications ranging from climate science to food safety, the instruments used to measure it are of utmost importance. Understanding these instruments allows professionals to ensure precision and reliability in their measurements. By exploring the diverse range of devices, their accuracy, and their specific uses, this article provides insight into the significance of temperature measurement in various contexts.
Research Overview
Summary of Key Findings
Various instruments exist for measuring temperature, each with unique operational principles and applications. Among traditional devices, the mercury thermometer is well-known, offering simplicity and ease of use, yet it has limitations regarding its environmental impact. The thermocouple, another common tool, operates on the principle of voltage generation due to temperature differences but often involves calibration complexities.
In contrast, modern technologies, such as infrared sensors and digital thermometers, have enhanced accuracy and ease of use. Infrared devices allow for non-contact measurements, making them suitable for sensitive applications, while digital thermometers provide quick readings with minimal user error. Advances in materials and technology continuously improve these instruments, pushing the boundaries of temperature measurement.
Significance of Research
The ongoing research into temperature measurement instruments holds significance across many fields including medicine, environmental monitoring, and industrial manufacturing. High-precision measurements lead to improved outcomes, whether in patient care through accurate body temperature readings or in quality control processes within manufacturing environments.
Understanding the limitations and capabilities of various measuring devices empowers professionals to select the most appropriate tools for their specific needs. This article aims to elucidate the landscape of temperature measurement instruments, considering their practical applications, technological advancements, and the evolving requirements of various industries.
Methodology
Research Design
This investigation employs a qualitative approach, analyzing historical data, contemporary studies, and case examples of different temperature measurement instruments. By looking closely at their efficacy, the research assesses how changes in technology have influenced their development and usage in diverse fields.
Data Collection Methods
Data for the analysis was collected through various methods:
- Literature Review: Academic publications and industry reports were reviewed to provide a comprehensive understanding of each instrument's functionality, advantages, and disadvantages.
- Case Studies: Specific instances of temperature measurements in various sectors, such as healthcare and the food industry, were examined to demonstrate real-world applications.
- Surveys and Interviews: Feedback from professionals who use these instruments enhanced the understanding of their practical challenges and benefits.
End
In closing, comprehending the array of instruments utilized for measuring temperature is essential for both academic study and practical application. This narrative seeks to offer a robust overview, enhancing the reader's ability to make informed decisions on instrument selection and application.
Preface to Temperature Measurement Instruments
Understanding temperature measurement instruments is essential. These devices are vital across various fields such as medicine, industry, and environmental science. Their ability to accurately measure temperature is key to ensuring safety, effectiveness, and quality in numerous applications. Temperature influences chemical reactions, biological processes, and physical states of matter. Therefore, precise measurement can mean the difference between success and failure in many scenarios.
Importance of Temperature Measurement
The importance of temperature measurement cannot be overstated. In medical settings, accurate temperature readings can indicate health issues or the effectiveness of treatments. For instance, a fever can signal an underlying infection. In industrial processes, temperature control affects product quality and machinery efficiency. Without proper measurement, products may fail safety standards or regulatory compliance. Likewise, in environmental monitoring, temperature data is crucial for assessing climate change impacts. Overall, temperature measurement serves as a foundation for decision-making in various sectors, enhancing both productivity and safety.
Historical Context
Historically, the quest for accurate temperature measurement has evolved significantly. Early civilizations used rudimentary methods, relying on the expansion of air or liquids in simple containers. By the 16th century, Galileo Galilei invented the first thermoscope, paving the way for more sophisticated devices. Later developments included the mercury thermometer, designed by Daniel Gabriel Fahrenheit in the early 18th century. This innovation greatly improved temperature accuracy with the use of mercury’s predictable expansion. Over time, new technologies emerged, leading to devices like thermocouples and infrared sensors, adaptable for diverse environments. Understanding this historical backdrop highlights how temperature measurement has transformed, laying the groundwork for contemporary methodologies.
Basic Principles of Temperature Measurement
Understanding the basic principles of temperature measurement is essential for anyone involved in scientific, industrial, or medical fields. Knowledge of these principles aids in selecting the right instrument for different applications, ensuring accurate temperature readings. Each principle explores a physical property related to temperature, which reflects changes in the environment or materials being measured.
Thermal Expansion
Thermal expansion refers to the increase in a material's dimensions as temperature rises. This principle operates on the premise that most substances expand when heated. In traditional thermometers, such as mercury and alcohol thermometers, thermal expansion is harnessed to provide a visual representation of temperature changes.
As the temperature increases, the liquid inside the thermometer expands and rises in a calibrated glass tube, allowing easy reading of temperature. The relationship between temperature and expansion is a linear one for small temperature ranges, making it straightforward to create accurate thermometers based on these principles. Moreover, understanding thermal expansion is crucial in engineering applications where structures and materials must accommodate the physical changes that arise from temperature fluctuations.
Thermal Radiation
Thermal radiation is the emission of electromagnetic waves from all matter that has a temperature above absolute zero. This principle is fundamental to infrared thermometers, which measure temperature from a distance. By detecting the infrared radiation emitted by an object, these instruments can provide accurate temperature readings without direct contact.
This principle plays a significant role in many applications, such as monitoring human body temperature or assessing the thermal performance of buildings. Furthermore, understanding the characteristics of thermal radiation allows for better insulation and energy efficiency designs in construction and manufacturing.
Electrical Resistance
The principle of electrical resistance is based on the observation that the electrical resistance of certain materials changes with temperature. Resistance Temperature Detectors (RTDs) and thermistors utilize this principle to give highly accurate temperature measurements.
RTDs operate through the predictable behavior of metals, such as platinum, where resistance increases as temperature rises. On the other hand, thermistors are semiconductor devices that exhibit a significant change in resistance with small temperature shifts. This principle's versatility makes it essential in areas like HVAC systems, where precise temperature regulation is crucial.
Phase Change
Phase change refers to the transition of a material from one state of matter to another due to temperature variation. This principle is prominent in devices such as the thermocouple, as well as in the use of melting point materials in calibration standards.
For instance, certain substances transition from solid to liquid at fixed temperatures, providing an accurate reference point. This reliability of phase change makes it advantageous for use in environments requiring precise calibration, such as laboratories. Understanding phase change properties is also useful in materials science and engineering applications where temperature-induced changes impact material integrity.
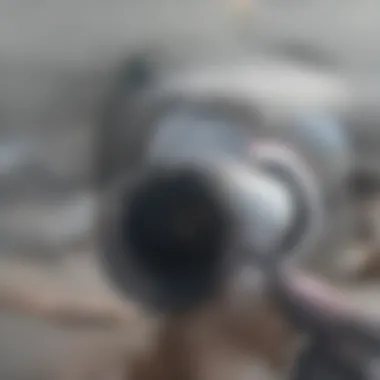
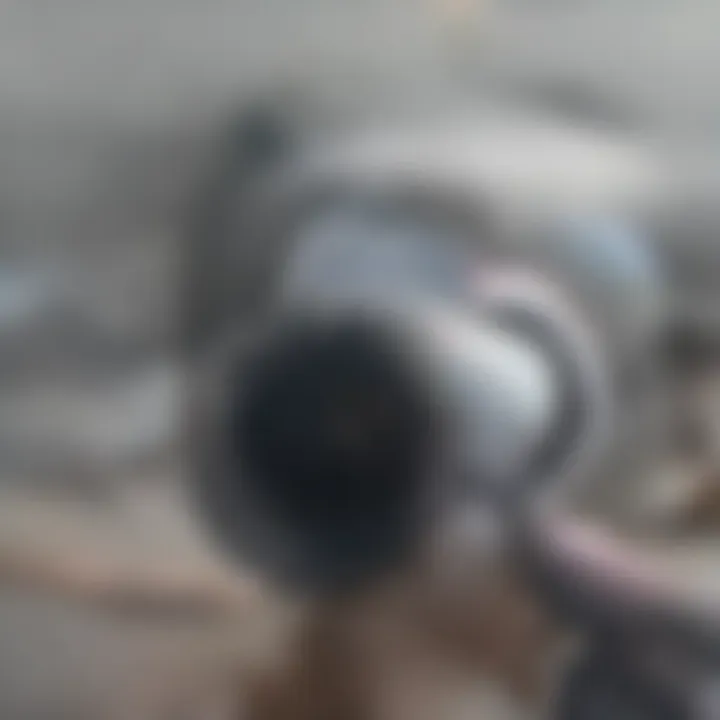
Incorporating these basic principles into the development and selection of temperature measurement instruments helps enhance accuracy and trustworthiness in readings. This understanding lays the groundwork for further exploration into traditional and modern technologies utilized in various applications.
Traditional Temperature Measurement Instruments
The topic of Traditional Temperature Measurement Instruments is vital for understanding the historical and foundational methods of measuring temperature. These instruments laid the groundwork for modern temperature measurement technology and showcase the evolution of scientific techniques. They are still relevant today in various applications due to their reliability, simplicity, and cost-effectiveness. Each of these instruments operates on different principles and has unique benefits to offer, facilitating diverse applications across many fields.
Mercury Thermometers
Mercury thermometers are perhaps the most recognized traditional instruments for measuring temperature. They operate based on the principle of thermal expansion. As temperatures rise, mercury expands and moves up the glass tube, providing a clear reading against a calibrated scale. This direct visual representation allows for easy interpretation of the readings.
Benefits of Mercury Thermometers:
- Accuracy: They provide precise readings, making them suitable for laboratory environments.
- Durability: When handled with care, mercury thermometers can be very long-lasting.
- Calibration: These thermometers can be regularly calibrated for maintaining accuracy.
However, the use of mercury has come under scrutiny due to its toxicity and environmental impact. Many regions have phased out mercury thermometers in favor of safer alternatives.
Alcohol Thermometers
Alcohol thermometers are an effective alternative to mercury thermometers. They use colored alcohol instead of mercury, which presents a safer option. Similar to mercury thermometers, alcohol thermometers measure temperature based on thermal expansion. Alcohol-based systems can also endure colder temperatures without freezing.
Key advantages include:
- Safety: They avoid the hazards associated with mercury, thus being environmentally friendly.
- Versatility: Ideal for both high and low-temperature measurements.
- Cost-Effectiveness: Generally more affordable than mercury thermometers.
On the downside, they can be less accurate at extreme temperatures and may require recalibration more frequently.
Bimetallic Thermometers
Bimetallic thermometers are mechanical devices that utilize two different metals bonded together. As temperature changes, the different rates of expansion of the metals cause the strip to bend, activating a pointer that indicates the temperature on a scale.
Advantages of Bimetallic Thermometers:
- Ruggedness: They are sturdy and do not contain liquids, reducing the risk of breakage.
- Suitability for Industrial Use: Ideal for environments with high temperatures and pressures.
- Ease of Reading: With a direct mechanical readout, these thermometers allow for quick assessments.
However, they may not provide the same level of precision as other methods and can be slow to respond to rapid temperature changes.
Gas Thermometers
Gas thermometers represent an earlier method of measurement that relies on the principle of gas expansion. A gas is contained within a bulb and tube system; as the temperature changes, the gas expands or contracts, causing movement in the liquid column, usually mercury, which indicates the temperature.
Benefits of Gas Thermometers:
- Wide Range of Measurement: They can be used to measure a broad temperature spectrum, including very low temperatures.
- Precision at Low Temperatures: Especially effective in cryogenic applications.
Despite their usefulness, gas thermometers can be cumbersome and require careful calibration and maintenance to ensure accuracy.
In summary, traditional temperature measurement instruments like mercury, alcohol, bimetallic, and gas thermometers have played a significant role in the field of temperature measurement. Their unique principles of operation and benefits highlight the legacy they contribute to today’s measurement techniques. Understanding these instruments offers valuable insight into the evolution of temperature measurement technologies.
Modern Temperature Measurement Technologies
Modern temperature measurement technologies play a pivotal role in achieving accurate and efficient temperature readings across various sectors. The rapid advancement in technology has led to the development of innovative instruments that enhance not only accuracy but also the speed of measurements. This section discusses four main technologies that have transformed temperature monitoring, including digital thermometers, infrared thermometers, thermocouples, and resistance temperature detectors. Understanding these instruments allows professionals to select the best options for their specific needs, thereby improving both research outcomes and industrial processes.
Digital Thermometers
Digital thermometers provide quick and accurate temperature readings. Unlike their analog counterparts, they display the temperature numerically, eliminating any ambiguity associated with reading a scale. These devices use sensors, commonly thermistors or semiconductor chips, to measure temperature changes. Speed is one of the key advantages of digital thermometers; they typically give readings within seconds. Also, they often feature additional functionalities, such as memory recall, alarm settings, and capability for data logging.
However, digital thermometers may have limitations in extreme conditions. Using them in environments with high temperatures or humidity may yield inaccurate results. Moreover, battery dependence is a concern, as it can limit usability if the batteries deplete. Despite these issues, their ease of use and digital interface make them a popular choice for home and medical purposes.
Infrared Thermometers
Infrared thermometers are unique instruments that measure temperature from a distance. By detecting the infrared radiation emitted by an object, these thermometers provide non-contact measurements, which is a considerable advantage in many scenarios where traditional measurement is impractical. This feature makes them especially valuable in cooking, industrial applications, and safety measures, where contact could be hazardous.
While they are highly effective for quick assessments, infrared thermometers can be influenced by emissivity. The surface texture and color of the object can affect the readings, requiring careful calibration or adjustments. Despite these considerations, the convenience and speed of infrared thermometers solidify their position in modern temperature measurement technologies.
Thermocouples
Thermocouples consist of two different metals connected at one end. When exposed to temperature gradients, they generate a voltage that correlates to the temperature difference. This feature allows for a wide range of temperature readings, making thermocouples suitable for diverse applications, especially in industrial settings. Their robustness and durability enable them to function efficiently in extreme temperatures and harsh environments.
Types of Thermocouples
Thermocouples come in various types, each designated by a specific configuration of metals. For example, Type K thermocouples are made of nickel-chromium and nickel-alumel, capable of measuring a wide range from -200°C to 1260°C. Their versatility, combined with relatively low cost, contributes to their popularity across different sectors.
The key characteristic of thermocouples is their fast response time, which allows for real-time monitoring of temperature changes. However, their accuracy may vary depending on the type and quality of the thermocouple used.
"Thermocouples are favored for their versatility in industry and wide measurement range."
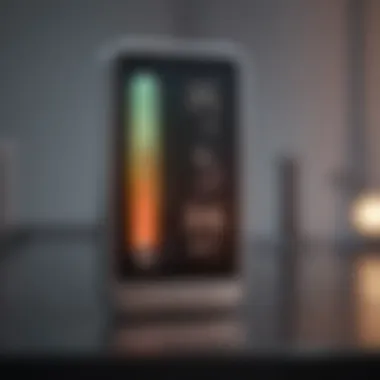
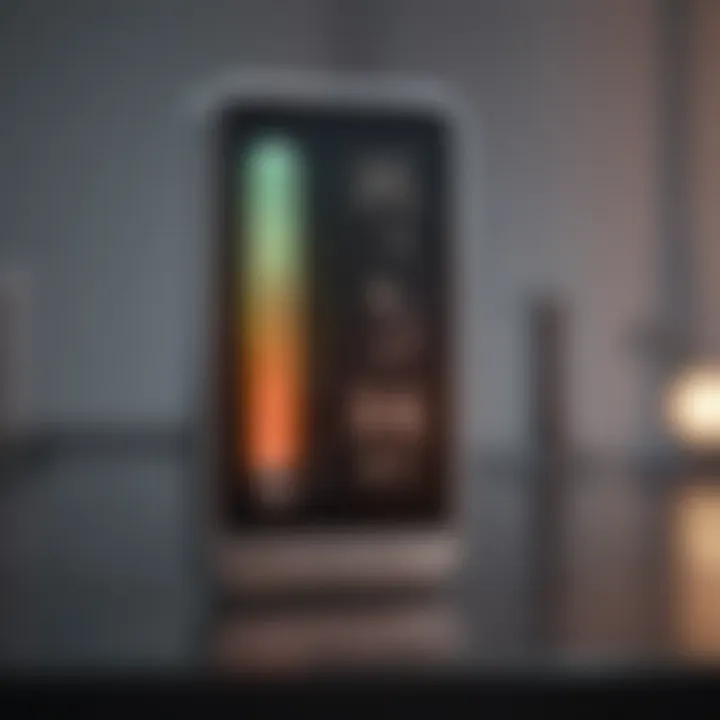
Applications in Industry
Thermocouples find extensive applications in industrial environments. From monitoring temperatures in furnaces to food processing, their robustness makes them an appropriate choice. They provide critical data for safety and operational efficiency by allowing continuous temperature monitoring in processes that require precise control.
Key features include their ability to function in adverse conditions and high-temperature environments. However, calibration is essential for maintaining accuracy, especially over time as materials may degrade or drift. This requirement can be a drawback in certain applications where maintenance is limited.
Resistance Temperature Detectors (RTDs)
Resistance Temperature Detectors, or RTDs, utilize the principle that the resistance of certain materials changes with temperature. Typically made with platinum, RTDs deliver more accurate and stable readings than thermocouples over specific temperature ranges. They excel in laboratory settings and industrial processes that demand precision.
One of the primary characteristics of RTDs is their linear response over a temperature range, making calibration more straightforward. However, they can be more expensive than thermocouples, which might deter some users.
Applications of Temperature Measurement
Understanding the applications of temperature measurement is crucial across various domains including healthcare, industry, and environmental sciences. The accurate measurement of temperature allows for essential decision-making processes, enhancing both safety and effectiveness. From diagnosing illnesses to ensuring the quality of products, temperature measurement proves its importance by delivering reliable data that informs practices and operations.
Medical Applications
In medicine, temperature measurement plays a pivotal role. Healthcare professionals use devices such as digital thermometers and infrared sensors to monitor patients’ body temperatures regularly. This practice aids in the diagnosis and management of conditions such as fevers, infections, and other health-related issues. The accuracy of these instruments is critical as even slight deviations can indicate serious health problems.
Moreover, in clinical settings, temperature regulation is vital during surgeries and in neonatal care. For instance, during operations, maintaining a patient's core temperature is essential to prevent hypothermia. Similarly, incubators for premature infants utilize precise temperature control to support their development. Thus, the reliance on accurate temperature measurement directly impacts patient outcomes.
Industrial Applications
In industrial contexts, temperature measurement is essential for process control, ensuring quality, and maintaining safety. Manufacturing processes in sectors such as food and beverage, pharmaceuticals, and chemical production require strict temperature regulations. A malfunction in temperature control can lead to defective products, which in turn may result in significant financial losses.
For example, in the food industry, monitoring cooking and storage temperatures is fundamental for food safety. Instruments such as thermocouples and resistance temperature detectors (RTDs) are commonly used for their reliability and precision in demanding environments. Such measurements not only uphold regulatory compliance but also protect consumer health.
Environmental Monitoring
Temperature measurement extends its significance to environmental monitoring as well. Climate studies rely on accurate temperature data collected from various sources like satellite sensors and ground stations. This information is vital for understanding climate change and its effects on ecosystems.
Additionally, accurate temperature readings in weather forecasting help predict extreme weather conditions, thereby enabling timely responses to natural disasters. Furthermore, in conservation efforts, monitoring the temperature of habitats can inform strategies to protect endangered species.
In all these applications—medical, industrial, and environmental—professionals must rely on accurate devices to ensure effective outcomes. By investing in advanced temperature measurement instruments, the integration of accuracy in processes becomes not just desirable but essential.
Accuracy and Precision in Temperature Measurement
In the realm of temperature measurement, accuracy and precision are pivotal concepts that determine the reliability and effectiveness of various measuring instruments. These terms, though often used interchangeably, possess distinct meanings that are essential in understanding how temperature measurements can impact scientific research, industrial processes, and even everyday applications. Accuracy refers to how close a measured value is to the true value, while precision indicates the reproducibility of the measurements under the same conditions. Both elements are fundamental to ensuring that temperature data is usable and trustworthy.
A high level of accuracy ensures that the values derived from temperature readings genuinely reflect the conditions being studied. In scientific experiments, where slight temperature variations can affect results significantly, accurate readings are essential. Determine whether the instrument detects slight changes can lead to discoveries or, conversely, to erroneous conclusions. Similarly, in industrial settings, such as food processing or pharmaceuticals, maintaining specific temperature ranges is crucial for product safety and efficacy. An inaccurate thermometer could have serious ramifications for quality control and regulatory compliance.
Precision complements this by indicating whether repeated measurements yield consistent results. High precision implies that multiple readings will cluster close together, irrespective of whether they approximate the true value. Instruments that provide high precision are vital when tracking changes over time, such as monitoring ambient temperatures or the thermal profiles of manufacturing processes.
Ultimately, understanding the nuances of accuracy and precision in temperature measurement can significantly contribute to enhancing research validity, improving process efficiencies, and ensuring regulatory standards are met.
Factors Affecting Accuracy
A variety of factors can influence the accuracy of temperature measurement instruments. Here are some key contributors:
- Calibration: Regular calibration against standardized references is essential to maintain accuracy. Instruments used in critical environments often require frequent checks and adjustments.
- Environmental Conditions: Surrounding temperature, humidity, and pressure may affect readings. For instance, thermocouples can exhibit varied responses in extreme conditions.
- Quality of Materials: The materials used in manufacturing thermometers can enhance or hinder accuracy. High-quality sensors often deliver better results than inferior ones.
- User Handling: Improper handling, such as incorrect positioning or exposure to sudden temperature changes, can result in flawed readings.
Calibration Procedures
Calibration is a systematic process designed to adjust the measuring instrument to ensure it provides accurate readings according to defined standards. Regular calibration should be conducted following a specific procedure:
- Selection of Calibration Standards: Utilize high-precision reference instruments that are traceable to national or international standards.
- Temperature Range Verification: Check the instrument across its entire range of operation to identify any inconsistencies.
- Establish Baseline: Record initial readings from both the standard and the instrument under test to create a baseline for comparison.
- Adjustment: If there are discrepancies, adjust the instrument as per manufacturer guidelines to correct any offset.
- Documentation: Maintain meticulous records of calibration dates, adjustments made, and conditions under which testing was performed.
Following detailed and rigorous calibration procedures helps ensure that temperature measurements remain reliable for their intended applications. This is vital for research environments where precise data can significantly influence outcomes.
Challenges and Limitations
Understanding the challenges and limitations of temperature measurement instruments is critical. These instruments, across their various designs and technologies, face certain constraints that can affect their performance, accuracy, and applicability in specific scenarios. A thorough grasp of these aspects can guide users in selecting the appropriate device for their needs and understanding the potential consequences of their limitations. The analysis of these challenges also encourages continuous improvement and innovation in the field of temperature measurement.
Material Limitations
Material limitations refer to the characteristics of the substances used in the construction of temperature measurement instruments. Each type of thermometer or sensor relies on specific materials to function correctly. For example, mercury in traditional thermometers is ideal due to its uniform thermal expansion, but hazards related to mercury toxicity have led to its gradual phase-out in many applications. Similarly, infrared thermometers depend on lenses made from specialized materials that must withstand environmental pressures while maintaining their optical properties.
In many cases, the choice of material directly affects the range and accuracy of the measurement. For instance, thermocouples must use metals that have predictable thermal properties. If the materials degrade or do not perform as expected, the results can be misleading, affecting reliability.
- Key Considerations:
- Compatibility with measured environments.
- Degradation of materials over time.
- Length of the measurement range.
Each of these points must be considered when selecting an instrument for specific applications, such as industrial settings or harsh conditions.
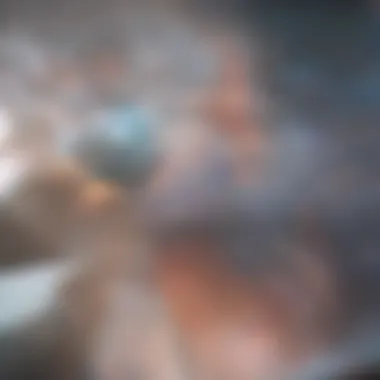
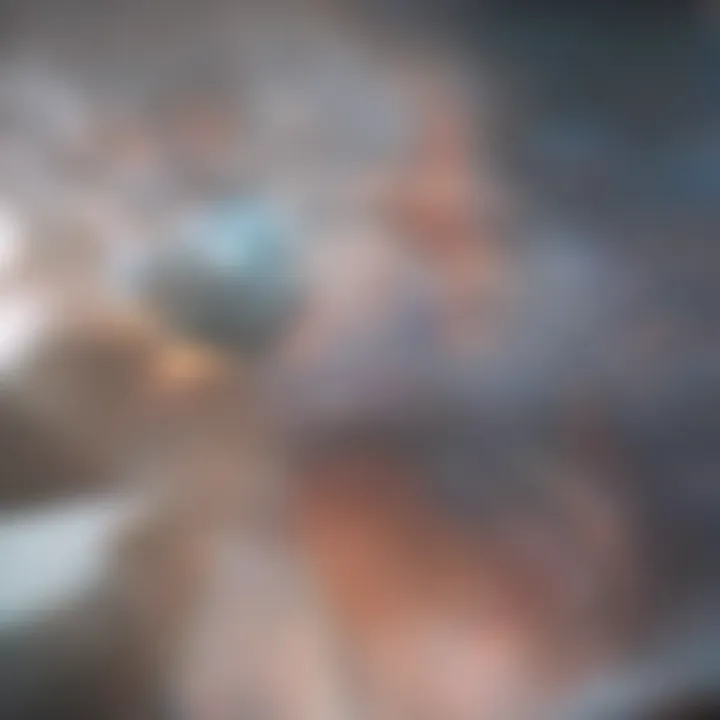
Environmental Factors
Environmental factors significantly influence temperature measurements. Devices may operate differently based on conditions such as humidity, pressure, and external temperature fluctuations. For instance, infrared thermometers can yield inaccurate readings if they are not calibrated properly for the object’s emissivity or are influenced by surrounding temperatures.
Moreover, some thermometric technologies can be slowed or affected by changes in surrounding environmental conditions. Specifically, digital thermometers may respond more slowly in extreme temperatures, whereas bimetallic sensors are mechanically reliant on physical changes and can have limitations in responsiveness.
- Critical Environmental Considerations:
- Humidity: Affects the accuracy of readings in certain devices.
- Pressure: Can alter the performance of gas thermometers.
- Surrounding Temperature: Influences infrared sensors negatively if not calibrated diligently.
"Understanding the interplay between the instrument and its environment is essential for obtaining reliable temperature data."
These facets underscore the importance of recognizing and anticipating environmental variables when employing temperature measurement instruments.
Innovations in Temperature Measurement
Temperature measurement is a critical aspect of various scientific and industrial applications. In recent years, innovations in temperature measurement technology have significantly enhanced the accuracy, efficiency, and applicability of these instruments. This section delves into the specific elements of emerging technologies and their integration into modern smart systems. Understanding these innovations is essential for professionals and researchers as they navigate the changing landscape of temperature measurement.
Emerging Technologies
Emerging technologies in temperature measurement are transforming the capabilities of instruments used in both laboratory and field settings. These advancements focus on improving precision, reducing response times, and expanding the operational range of temperature measurement devices.
- Wireless Temperature Sensors: These sensors allow for remote monitoring of temperature, eliminating the need for physical access. This is especially beneficial in environments that are hazardous or difficult to reach.
- Microelectromechanical Systems (MEMS): MEMS technology enables the development of smaller and more sensitive temperature sensors. They can be incorporated into compact devices, allowing for innovative applications in wearable technology and medical diagnostics.
- Nanotechnology: Nanoscale materials have unique thermal properties. Using nanotechnology in temperature sensors can improve their responsiveness and sensitivity, making them suitable for applications where traditional methods fall short.
- Optical Temperature Sensors: These sensors use light to measure temperature and are less susceptible to interference than traditional electronic sensors. This technology is valuable in high-voltage environments.
Emerging technologies enhance how temperature measurement is approached in various sectors, highlighting the need for continuous research and development.
Integration with Smart Systems
The integration of temperature measurement instruments with smart systems is another significant innovation. Smart systems leverage the Internet of Things (IoT) to facilitate real-time monitoring and data analysis, enhancing the overall efficiency of processes.
- IoT-Enabled Devices: Temperature measurement devices now come equipped with IoT capabilities. This integration allows for automatic data collection and cloud connectivity, enabling users to monitor temperature changes remotely.
- Data Analytics: With real-time data collection, advanced analytics can be applied to regulate processes. For example, industries can predict maintenance needs based on temperature trends, thus optimizing production schedules.
- Automation: Integration with smart systems leads to increased automation in temperature control processes. Automated systems can adjust heating and cooling mechanisms based on real-time temperature readings, improving energy efficiency.
- Enhanced User Interface: Many modern temperature sensors now feature user-friendly interfaces, allowing for easier interaction and data interpretation. This is crucial for industries where quick decision-making is necessary.
"The synergy of temperature measurement instruments with smart technologies is reshaping operational efficiency across sectors."
The innovations in temperature measurement and their integration into smart systems represent a crucial evolution in how temperature data is collected, analyzed, and applied. Staying updated with these advancements is key for professionals and researchers who aim to optimize their processes and gain deeper insights into temperature management.
Future Trends in Temperature Measurement
The landscape of temperature measurement is evolving at a rapid pace. Understanding the future trends of this field is critical not only for academic advancements but also for practical applications across various industries. As technologies advance, the integration of smart solutions and enhanced sensor technologies is becoming paramount. This evolution promises greater accuracy, efficiency, and broader applications of temperature measurement instruments.
Advancements in Sensor Technologies
Recently, sensor technologies have experienced significant advancements. These improvements stem from innovations in materials and design. New sensor types, like nanomaterial-based sensors, have begun to emerge. These sensors exhibit notable sensitivity and can detect even the slightest temperature changes. Moreover, wireless sensor networks are becoming commonplace. They permit remote temperature monitoring, which is invaluable in process control and environmental studies.
Key aspects of advancements include:
- Miniaturization: Smaller sensors allow for integration in a variety of devices, enhancing their versatility.
- Enhanced Sensitivity: Improved materials lead to sensors that detect a wider range of temperatures with high precision.
- Real-time Monitoring: Technology allows continuous tracking, providing immediate data that can be critical for decision-making.
These advancements in sensor technologies have ramifications across scientific research, medicine, and industrial applications. These systems assure both reliability and accuracy, providing essential data for ongoing analysis and operations.
Applications in Emerging Fields
Temperature measurement plays an increasing role in new and evolving sectors. Innovations in the field are pushing the boundaries of where and how temperature sensors are utilized. Notably, fields such as IoT (Internet of Things), agriculture, and biotechnology are showing substantial promise.
In IoT, temperature sensors can be connected to a network for data collection, enabling smart home systems and industrial automation. They help in monitoring conditions in real-time, promoting energy efficiency and safety.
In agriculture, precision farming techniques utilize temperature sensors to monitor soil and air conditions, helping farmers make informed decisions based on accurate data that can enhance crop yield.
The increasing complexity and sophistication of biotechnology also depend heavily on temperature regulation. In processes such as fermentation or drug storage, maintaining precise temperatures is vital for success. Sensors that can operate under extreme conditions or in sterile environments are in demand.
The trends mentioned signify a shift in how we understand and apply temperature measurement instruments. As fields continue to advance, the implications for research, industry, and practical applications become more apparent.
The End
The conclusion section serves as a critical summation of the comprehensive overview on temperature measurement instruments. It pulls together the diverse threads explored in the article, reinforcing the essential role these instruments play in various fields. Recognizing the importance of temperature measurement offers key insights into scientific research, industrial applications, and everyday life.
Recapitulation of Instruments
Throughout the article, a multitude of temperature measurement instruments have been discussed. These range from traditional devices like mercury and alcohol thermometers to modern innovations such as digital thermometers and infrared sensors. Each instrument operates on distinct principles and exhibits specific advantages and limitations, recognizing their suitability for different applications.
- Mercury Thermometers: Historically significant and still used, despite safety concerns.
- Alcohol Thermometers: Alternative to mercury; safe for many applications.
- Digital Thermometers: Fast and accurate, common in medical fields.
- Infrared Thermometers: Non-contact measurements, useful in various industries.
- Thermocouples and RTDs: Essential for industrial temperature monitoring and precision tasks.
This diversity highlights the need for practitioners to select the right instrument based on specific requirements, ensuring accuracy and reliability in their measurements.
Implications for Research and Industry
The implications of accurate temperature measurement extend far beyond mere number recording. In research environments, precision influences experimental outcomes. Industries rely on temperature control for efficient operations, safety, and compliance.
- Quality Control: Temperature monitoring is vital in processes such as food production and pharmaceuticals, ensuring that products meet safety standards.
- Environmental Science: Accurate measurements assist in climate studies, biology research, and ecological monitoring, influencing policy and conservation efforts.
- Healthcare: In medicine, careful temperature regulation can be the difference between success and failure in treatment.
Considerations for future developments indicate a trend toward smart systems integration. Emerging technologies promise improved performance and usability, emphasizing the ongoing evolution in temperature measurement methodologies.