In-Depth Analysis of Brain Lab Research Protocols
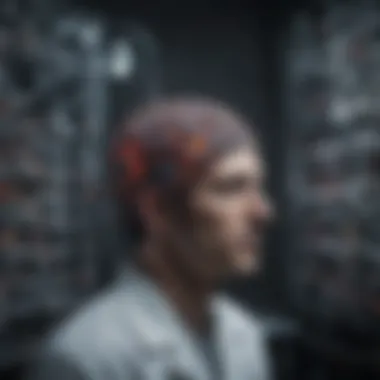
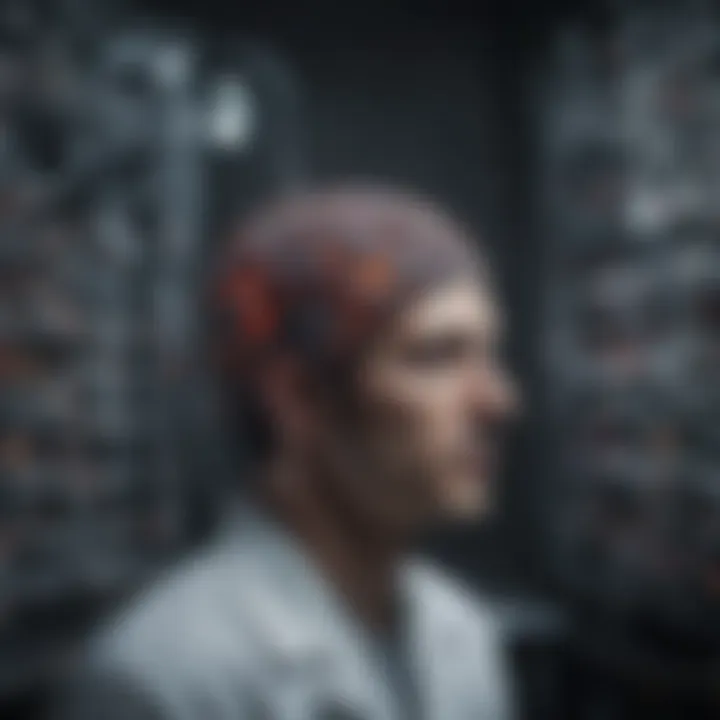
Intro
When delving into the complexities of neuroscience, one must navigate a labyrinth of protocols and methodologies that form the backbone of research in brain laboratories. This exploration aims to shed light on the essential aspects of brain lab protocols, underscoring their significance not just in academic realms but across various applications in society.
As any seasoned researcher would know, the study of the brain presents a myriad of challenges — from ethical considerations to technological limitations. Neuroscience isn’t simply about gathering data; it’s about understanding the complexities of life itself. This article draws back the curtain on the multifaceted world of brain lab protocols, offering insights into every nook and cranny of research practices that adhere to established guidelines.
Research Overview
Summary of Key Findings
In this section, we take stock of the prevailing knowledge in the field of brain research. Notably, significant advancements have been made in areas such as neuroimaging techniques, which now afford researchers a non-invasive view of the brain. This has transformed our understanding of conditions ranging from Alzheimer's disease to epilepsy.
Moreover, data processing methodologies have become increasingly sophisticated, allowing for high-resolution insights into neural activity and connectivity. By systematically analyzing brain signals, researchers can now uncover relationships that were previously veiled in mystery. Noteworthy findings share insights on neuroplasticity and its implications for rehabilitation following brain injuries, which are profoundly affecting therapeutic strategies.
Significance of Research
Understanding brain lab protocols is about more than research; it reflects an evolving landscape of ethical and practical implications. The principles governing these protocols ensure that research is conducted respectfully and responsibly. They demand rigor, accountability, and vigilance, especially given the sensitive nature of studies involving human subjects. As such, the significance extends into how results influence patient care, policy-making, and public understanding of neurological health.
"The meticulous adherence to established protocols is not merely a procedural formality; it embodies a commitment to the integrity and advancement of neuroscience."
That's a thought worth pondering, especially when considering the potential ramifications of research findings. In an age when misinformation can easily proliferate, grounded research practices provide a beacon of reliability to guide further inquiries.
Methodology
Research Design
The design of research in brain labs varies greatly depending on the specific questions posed. Generally, designs can be classified as observational or experimental. Observational studies allow researchers to witness phenomena as they unfold in real time, often gathering insight from clinical populations. Experimental designs, on the other hand, enable controlled tests that can reveal cause-and-effect relationships.
A well-structured research design begins with a thorough literature review. This step is crucial in identifying gaps in knowledge that the intended study will aim to fill. Following this, a hypotheses-driven approach forms the foundation for data collection and analysis methods.
Data Collection Methods
Data collection methods in brain labs are diverse and often depend on technological capabilities. Common methods include:
- Functional Magnetic Resonance Imaging (fMRI): This technique measures brain activity by detecting changes in blood flow, giving insights into specific brain functions during tasks.
- Electroencephalography (EEG): This method records electrical activity along the scalp, providing high temporal resolution, which is useful for tracking brain responses over time.
- Magnetoencephalography (MEG): Similar to EEG, but measures the magnetic fields produced by neural activity, allowing for enhanced spatial resolution.
- Animal studies: These provide vital data by examining the brain structures and functions of non-human subjects, contributing to our understanding of complex behaviors.
In summary, the protocols governing brain lab research are designed not only to maintain scientific integrity but also to illuminate the profound mysteries of the human brain. This guide serves as a comprehensive resource for academics, researchers, and professionals alike — all key players in the ongoing endeavor to unlock the secrets held within our minds.
Foreword to Brain Lab Protocols
Understanding brain lab protocols is crucial in neuroscientific research. This article sheds light on the structured methodologies that guide researchers in the exploration of brain functions and their underlying mechanisms. Protocols serve as blueprints, ensuring that experiments are conducted consistently and ethically while yielding reproducible results. Moreover, these guidelines help in maintaining safety standards which is paramount when dealing with living tissues or human subjects.
In a world where research can be the difference between life and death, adhering to proven protocols not only advances knowledge but also saves countless lives through applied science and medicine. With a solid grasp of these protocols, researchers can optimize their inquiries, making significant discoveries that push the boundaries of what we know about the brain.
Definition and Purpose
Brain lab protocols refer to a set of structured guidelines that dictate the methodologies employed in neuroscientific research. These protocols are designed to facilitate not only data collection and analysis but also to ensure ethical treatment of subjects, be they human or animal. The primary purpose is to create a framework that fosters consistency across experiments, makes findings comparable, and establishes a foundation for future research.
The protocols vary widely depending on the specific focus of the research. For instance, protocols for in vivo studies, which examine living organisms, are quite distinct from those designed for in vitro analyses, which take place in controlled environments outside a living organism. By defining clear parameters, these protocols reduce ambiguities and enhance the reliability of results, allowing researchers to build upon one another's work effectively.
Historical Context
The evolution of brain lab protocols traces back centuries, rooting itself in the development of science as a rigorous discipline. During the early days of neuroscience, research was often characterized by a lack of standardized methods. Trials and errors were commonplace, with findings frequently disputed due to varied experimental conditions.
In the late 19th and early 20th centuries, the advent of modern scientific techniques prompted the establishment of initial protocols. Pioneers, such as Santiago Ramón y Cajal and Ivan Pavlov, set the groundwork by insisting on reproducibility and methodological rigor in their work. The importance of ethics in research also gained traction during this era, leading to gradual changes in how studies involving human and animal subjects were conducted.
As neuroscience matured, particularly in the latter part of the 20th century, clear and formalized protocols came into existence. This shift allowed for enhanced collaboration across disciplines, from psychology to medical research. Consequently, institutions now prioritize protocol development, emphasizing the need for transparency and consistency in research practices. Going forward, staying attuned to historical progress informs how contemporary research is designed and executed, driving the field toward even greater heights.
Types of Brain Lab Protocols
Understanding the types of brain lab protocols is vital for anyone involved in neuroscientific research. These protocols significantly impact the effectiveness and applicability of experiments, thereby aiding in drawing reliable conclusions. This section divides the protocols into two main categories: in vivo and in vitro. Each category has its unique methodologies, applications, and specific challenges that researchers must navigate. Recognizing these elements can help professionals and students in tailoring their research approaches, optimizing resource utilization, and ensuring good practice in their experiments.
In Vivo Protocols
In vivo protocols refer to studies conducted on whole organisms. Such protocols are essential for observing the functioning of biological systems in real time, maintaining the complexities of behavior and biological interactions.
Methodologies
The methodologies of in vivo studies vary widely, ranging from behavioral assessments to advanced imaging techniques. Notably, in vivo imaging allows researchers to view dynamic biological processes, making it a favored method. It provides live insights into biological mechanisms without requiring invasive procedures, thus enabling longitudinal studies over time.
The tag of real-time observation sets in vivo methodologies apart. For instance, using functional MRI (fMRI) offers the advantage of non-invasively assessing brain activity via blood flow changes. However, there's a downside; interpreting fMRI data requires careful consideration to avoid misinterpretation of the neural correlates.
Applications
In vivo protocols are crucial for drug efficacy testing, neurodevelopment studies, and behavioral neuroscience. They allow for complex interactions to be studied within an intact organism, offering valuable insights that in vitro models can't provide. One significant application is in the study of neurodegenerative diseases; models such as transgenic mice are often used to replicate human conditions.
The key advantage of in vivo research lies in its ability to simulate natural conditions which is often absent in other research methods. However, ethical concerns regarding animal welfare and the complications associated with translational research must be taken into account.
Challenges
The challenges surrounding in vivo protocols often stem from ethical, technical, and biological variability aspects. First, ethical use of animal subjects raises questions about humane treatment and the appropriateness of experimental designs. Next, technical issues arise from the need for specialized equipment and the potential for equipment failure during critical experiments.
Finally, another significant hurdle is biological variability, which can lead to inconsistent results across different organisms. Addressing these issues is essential; transparency in methodologies can lead the way to developing harmonious relationships within the research community and regulatory bodies.
In Vitro Protocols
In vitro protocols, in contrast, take place outside of a living organism, typically in a controlled laboratory environment. This approach allows for high precision and control over experimental conditions when studying cellular and molecular processes.
Techniques Used
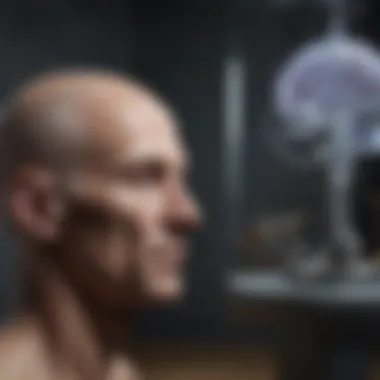
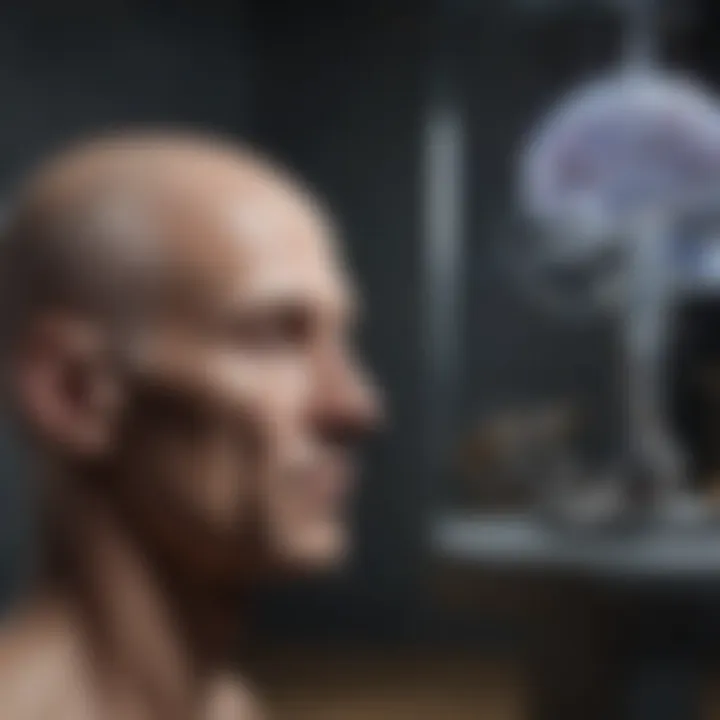
Common techniques used in in vitro protocols include cell culture, electrophysiological measurements, and molecular assays. The cell culture technique is foundational; it enables researchers to manipulate specific cellular environments, assess drug responses, and study cell signaling pathways without the organic variability present in whole organisms.
A standout feature of in vitro techniques is their potential to conduct experiments with high reproducibility. However, it should be noted that results derived from these studies might not always translate seamlessly into in vivo scenarios. The controlled environment can sometimes lead to oversimplified representations of complex biological processes.
Benefits and Limitations
One critical benefit of in vitro methods is their ability to provide high-throughput data collection, crucial for large-scale screening of drug candidates. They facilitate risk assessment without needing to rely on whole organism models, thus reducing ethical considerations for animal testing. Yet, the limitation lies in the inability to replicate the intricate interactions that occur within a living organism, leading to potential gaps in understanding.
In summary, both in vivo and in vitro protocols offer unique advantages and challenges. As technologies advance and ethical standards evolve, a blended approach may emerge, helping to bridge these gaps and foster a more integrated understanding of the brain's function.
Essential Components of Protocols
Understanding the essential components of brain lab protocols is fundamental for conducting reliable research in neuroscience. These components form the backbone of each protocol, ensuring that every experiment is repeatable, ethical, and scientifically valid. Navigating through various protocols, the significance of integrating robust components can’t be overstated, as they not only enhance the quality of the findings but also ensure compliance with ethical and regulatory standards.
Equipment and Technology
Imaging Tools
One of the most crucial aspects of any brain research protocol involves imaging tools. These devices permit the visualization of brain structures, enabling researchers to track changes over time. A key characteristic of imaging tools, like Magnetic Resonance Imaging (MRI) or Positron Emission Tomography (PET), is their ability to provide non-invasive insights into both the anatomical and functional aspects of the brain.
The popularity of MRI, for instance, arises not just from its detailed images but also from the fact that it poses minimal risk to participants compared to surgical procedures. One unique feature of MRI is its ability to distinguish between different types of tissues based on their water content and environmental factors. This makes it especially advantageous when assessing conditions like tumors or lesions. However, the disadvantages include longer acquisition times and expensive setup costs, which not every lab can afford.
Neurophysiological Instruments
Neurophysiological instruments play a vital role in capturing real-time data about brain activity. Tools like electroencephalograms (EEG) and magnetoencephalograms (MEG) are cornerstones in the assessment of neural dynamics. The key characteristic that sets neurophysiological instruments apart is their temporal resolution—they can record brain activity as it happens, allowing for a deeper understanding of cognitive processes.
EEG is particularly valued for its relatively low cost and portability, allowing researchers to conduct studies in diverse settings, even outside the lab. However, it suffers from limited spatial resolution, making it tricky to pinpoint the exact location of the brain activity recorded. MEG, while it offers better localization, carries a hefty price tag that may be beyond the means of some research institutions.
Sample Collection and Processing
Preparation Techniques
The preparation techniques used for collecting samples are decisive in research protocols. These procedures must be meticulously designed to ensure the integrity of the samples. A key characteristic of preparation techniques is their ability to minimize contamination and preserve the biological characteristics of the samples being studied. Techniques like cryopreservation and chemical fixation are utilized extensively to maintain neural tissue viability during experimentation.
Though cryopreservation is invaluable for its long-term viability of samples, it can be a complex process requiring specialized equipment and expertise. On the other hand, chemical fixation, while simpler, often alters the tissue in ways that can obscure certain biological properties, which can impact the results of the experiments.
Storage Conditions
Storage conditions are just as crucial as preparation techniques in brain research. Depending on the nature of the samples, specific conditions must be maintained to prevent degradation. An essential characteristic here is the stable temperature and humidity control that optimizes sample quality through storage.
Maintaining strict cold chain logistics is a popular choice for protocols dealing with biological samples, especially those sensitive to temperature changes. This can prolong the efficacy of samples for future analysis. However, the requirement for specialized freezers or storage environments can present logistical challenges and added costs.
"Essence of a solid brain lab protocol lies in its components—each is a cog in ensuring the wheels of research run smoothly."
In summary, the essential components of protocols encompass crucial elements like equipment, technologies, sample collection, and processing methods. The capability of each component to effectively contribute to the overarching research objectives makes their careful selection and application absolutely vital. Balancing benefits and limitations as researchers choose their components is a challenge—one that ultimately defines the quality of their findings.
Protocol Design and Implementation
Designing and implementing protocols is a backbone of scientific inquiry in brain labs. It systematically organizes the complex processes that researchers follow, ensuring both accuracy and reproducibility in their findings. Crafting a well-structured protocol not only streamlines methods but also minimizes errors that can arise in experimental settings. Moreover, a rigorously developed protocol fosters clarity, enabling researchers from varying backgrounds to replicate studies and advance the field collaboratively. This segment outlines the nuances in creating effective protocols and emphasizes the best practices in their execution.
Formulating a Protocol
Defining Objectives
Defining objectives is the cornerstone when formulating any protocol. It determines the purpose behind every experiment and guides the direction of the research efforts. By outlining clear and measurable goals, researchers can ensure that their methodologies align closely with their intended outcomes. A critical characteristic of defining objectives is its role in emphasizing specificity. This specificity helps in avoiding vague interpretations of results, which can lead to confusion later on.
An essential aspect is quantifying the objectives. Whether it’s measuring neuronal growth or assessing behavioral responses, having a target provides clarity. This has become a popular choice in brain lab settings because it holds researchers accountable for their expected outcomes. A unique feature of defining objectives is its adaptability; objectives can evolve as research progresses, allowing for more nuanced explorations. However, if objectives are too rigid, they may stifle exploration into interesting avenues that arise unexpectedly during research.
Selecting Approaches
Selecting appropriate approaches complements objective definition by determining how those objectives will be achieved. This involves evaluating various methodologies available, from electrophysiological techniques to neuroimaging strategies, ensuring that the chosen methods align with the existing objective framework. The key characteristic of selecting approaches is its multifaceted nature, as various methods can yield different insights. Many researchers find this beneficial since a diversified methodological toolkit can enhance the richness of data gathered.
One distinctive feature of this selection process is the careful consideration of equipment and reagent availability. Being aware of what tools are on hand influences the feasibility of certain approaches. An advantage of thoughtful method selection is it bridges the gap between theoretical understanding and practical application. However, it’s important to remember that some cutting-edge techniques may require extensive training or expertise, which could hinder progress depending on lab resources.
Standard Operating Procedures
Having standard operating procedures (SOPs) ensures consistency across experiments and fosters a culture of excellence in the lab. SOPs act as a living document that outlines accepted methods and protocols, providing a template for future studies. This uniformity ensures that everyone in the lab, regardless of experience level, operates under the same guidelines, which streamlines processes and reduces variability in results.
Documentation Practices
Documentation practices represent an integral aspect of SOPs. They encapsulate records that detail experimental conditions, equipment used, and observations made during trials. Ensuring thorough and meticulous documentation enhances the overall reliability of data. Documentation is vital as it allows for retrospective analyses and learning from past experiments. One notable characteristic is how it cultivates a shared knowledge base. By recording processes, researchers contribute to a body of work that can guide new members in the lab.
A unique feature of documentation practices is the adaptability they foster. Researchers can attach notes regarding unexpected outcomes or adjustments made to protocols. While comprehensive documentation is advantageous, the challenge lies in striking a balance between thoroughness and practicality. Excessive documentation may lead to redundancy or overwhelm, causing potential fatigue in researchers trying to navigate records.
Compliance with Regulations
Compliance with regulations safeguards ethical integrity within brain research, ensuring that studies align with established legal and ethical standards. This aspect of SOPs is crucial in fostering respect for experimental subjects and maintaining a high standard of scientific integrity. The importance of this compliance cannot be overstated; it not only protects researchers but also nurtures public trust in scientific research.
A key characteristic of compliance is its necessity for funding and publication. Many grants require adherence to specified guidelines, and journals may also stipulate compliance before considering manuscripts for publication. The unique aspect of regulatory compliance is that it varies across regions and institutions, making it essential for researchers to stay updated with the ever-evolving landscape of legislation and ethical standards.
Though compliance enhances the credibility of results, it can sometimes involve cumbersome paperwork that may delay research progress. Striking a balance here, researchers need to prioritize compliance while ensuring that it does not become an impediment to their investigative efforts.
"In the labyrinthine world of brain science, a well-formulated protocol acts as a flashlight, illuminating the path for future discoveries."
In summary, careful consideration of protocol design and implementation lies at the heart of reliable and successful brain research. By meticulously defining objectives, selecting appropriate approaches, and maintaining robust SOPs, researchers can optimize their practices, contributing to advancements within the field.
Ethical Considerations in Brain Research
Ethical considerations play a crucial role in brain research, shaping the very foundation upon which scientific inquiry is conducted. As we delve deeper into understanding the brain and its functions, the moral responsibilities that researchers hold cannot be overlooked. Upholding these ethical standards ensures not only the integrity of the research but also the welfare of the subjects involved, whether they be animals or humans. The discussion of ethical standards also goes hand in hand with the kind of trust that the research community needs to maintain with the public.
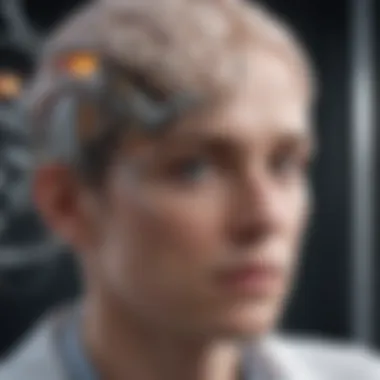
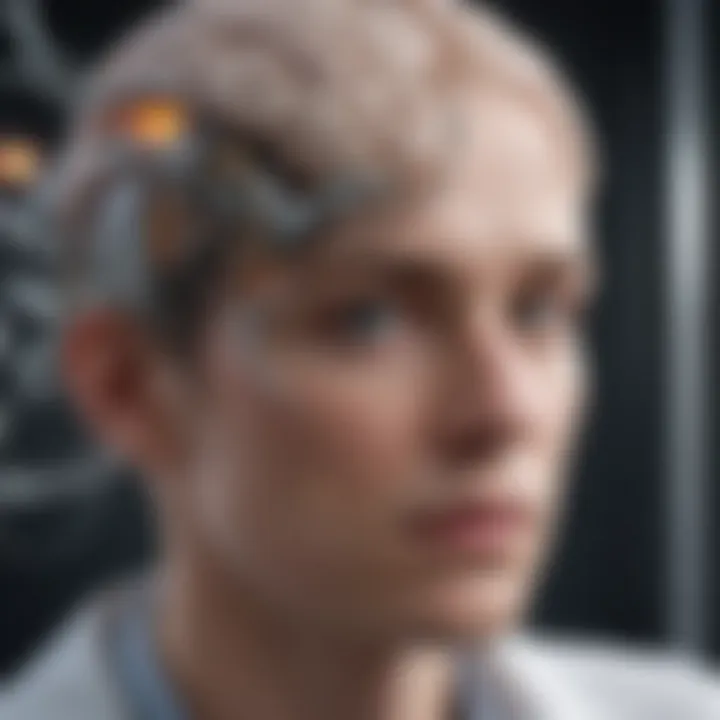
Animal Welfare
The concept of animal welfare is paramount when it comes to protocols in neuroscience. Researchers have an obligation to treat all animal subjects with respect and dignity, acknowledging their value beyond mere research tools. This involves ensuring that animals are kept in humane conditions and that their pain and distress are minimized. Ethical protocols are often guided by the principles of the 3Rs: Replacement, Reduction, and Refinement.
- Replacement refers to finding alternatives to the use of animals in research. This can mean using in vitro studies or computer models.
- Reduction involves using fewer animals to achieve the same scientific conclusions. Researchers can often analyze data more efficiently or opt for methods that require a smaller sample size.
- Refinement pertains to enhancing animal welfare by improving procedures or environments.
Implementing these principles doesn’t just comply with regulations; it elevates the quality of findings. A study conducted with care towards animal welfare likely yields more reliable and generalizable results, ultimately benefiting the field at large.
Human Subject Research
Human subject research raises its own ethical considerations, primarily rooted in respect for individual autonomy and welfare. This is especially evident in the processes of informed consent and risk assessment.
Informed Consent
Informed consent is a cornerstone of ethical human subject research. It involves providing potential participants with clear, concise information about the study’s purpose, procedures, risks, and benefits. Participants must voluntarily agree to partake, without coercion. This practice fosters trust and maintains transparency between researchers and participants.
A significant characteristic of informed consent is its adaptability; it should cater to the understanding of each individual. This involves breaking down complex medical jargon into simpler terms, allowing even those with limited education to grasp what they are signing up for. One unique feature of this consent process is its ability to empower participants. Not only does it legitimize their involvement, but it also fosters a sense of agency, making them feel more engaged in the research process.
Though some may argue that detailed consent forms can overwhelm potential subjects, the advantages far outweigh the disadvantages. Giving individuals comprehensive knowledge about their involvement in a study ultimately leads to better decisions, fortifying the ethical backbone of research efforts.
Risk Assessment
Risk assessment is another critical aspect that cannot be overlooked. It involves evaluating the potential physical, psychological, and emotional risks that participants might face during a study. Effective risk assessment allows researchers to take preemptive measures to ensure subject safety and well-being.
One key characteristic of risk assessment is its proactive nature; it prompts researchers to anticipate and mitigate risks before they occur. This foresight not only protects participants but also upholds the integrity of the research. Recognizing potential dangers can lead to modifications in the study design, resulting in better overall outcomes.
However, navigating the nuances of risk assessment can be challenging. Some researchers might understate risks, while others may err on the side of caution, inadvertently deterring participation. Yet, this careful balancing act is pivotal in fostering a research environment where participant safety comes first.
Ultimately, ethical considerations in brain research aren't just a box-tick exercise. Rather, they represent a commitment to practice science responsibly, ensuring that the quest for knowledge does not come at the expense of humane treatment.
Best Practices for Data Management
Data management in brain lab protocols is paramount for the success and reliability of research outcomes. The way data is collected, organized, and analyzed can make or break the reproducibility of a study. When protocols are solidly designed around data management best practices, researchers can ensure their findings are not only valid but also build on existing knowledge in the field.
Here are a few specific aspects to consider:
- Data Integrity: Ensuring the accuracy and consistency of data throughout the research process.
- Accessibility: Structuring data so it’s easy for other researchers to access, understand, and utilize.
- Security: Safeguarding sensitive information, especially when dealing with human subjects.
Data Collection Techniques
In brain research, data collection techniques can vary widely depending on the type of study. Common methods include:
- Surveys: Used extensively in psychology and related fields.
- Electrophysiological recordings: Essential for neurophysiological studies to capture real-time brain activity.
- Neuroimaging Techniques: Like fMRI and PET scans, allow for visualization of brain structures and functions.
The choice of technique often hinges on the research objectives and the type of data required. For instance, if the goal is to analyze neural responses to stimuli, electrophysiological methods will likely be preferred over more general approaches like surveys.
Analysis and Interpretation
Analysis of data can be complex, especially with large datasets typical in neuroscience. Two prominent aspects that often capture attention are statistical methods and data visualization.
Statistical Methods
Statistical methods are crucial in transforming raw data into meaningful insights. They help in quantifying relationships, making predictions, and drawing conclusions about the hypothesis being tested. A key characteristic of statistical methods is their ability to provide a structured way to evaluate uncertainties and variabilities in data.
One particularly beneficial statistical approach is the use of multivariate analysis, which allows researchers to understand how multiple variables interact. This is especially relevant in brain research where numerous factors can influence outcomes. The unique feature of employing such methods is their capacity to discern patterns that might be invisible to straightforward descriptive statistics.
However, these statistics can come with limitations. Misinterpretations often arise, particularly when researchers overlook the assumptions underlying some statistical tests. This can lead to erroneous conclusions, emphasizing the need for thorough understanding and careful application of selected methods.
Data Visualization
Data visualization serves as a powerful tool in interpreting and presenting complex datasets. The ability to turn raw numbers into graphical representations allows researchers and audiences to grasp the findings rapidly. One defining characteristic of effective data visualization is clarity; it should convey the essential message without causing confusion.
Utilizing techniques like heatmaps or brain atlases can unravel intricate neural interactions effectively. Moreover, visualization serves a dual purpose: it aids in data interpretation while also enhancing communication of findings to an audience, which could range from academics to policy-makers.
Nevertheless, while data visualization can significantly enhance understanding, it can also mislead if not designed properly. Overly complex charts may obscure the main thesis or introduce biases in interpretation. Thus, it's vital that scientists utilize these tools strategically, ensuring their representations truly reflect the data being analyzed.
The integrity of data management is not merely about safeguarding information; it's about cultivating a research culture built on transparency and collaboration.
Current Trends and Innovations
Recent years have witnessed a significant evolution in brain lab protocols, driven largely by technological advancements and interdisciplinary collaboration. These current trends not only enhance the methodologies used in neuroscience research but also provide profound insights that were previously unconceivable. By embracing these innovations, researchers can refine their practices, improve data accuracy, and open avenues for novel explorations into brain function and disorders.
Neuroimaging Advances
Neuroimaging continues to revolutionize the understanding of brain dynamics. Techniques such as functional magnetic resonance imaging (fMRI) and diffusion tensor imaging (DTI) have changed how researchers examine the brain's structure and activity. These methods not only allow for mapping brain pathways but they also help in assessing abnormalities associated with neurological disorders.
For instance, the application of real-time fMRI enables scientists to observe brain activity in response to various stimuli, which helps in understanding cognitive processes.
"With the rise of advanced imaging tools, we are venturing into territories that were once thought to be merely speculative in neuroscience."
This evolution has practical implications. With less invasive techniques, subjects experience reduced discomfort, leading to more natural engagement during studies. Moreover, new software designs that facilitate advanced analysis of neuroimaging data are becoming essential components in protocol design, allowing for a more nuanced interpretation of results.
Computational Neuroscience
Computational neuroscience serves as a bridge between theoretical models and practical experimentation in brain research. The combination of biology and computer science creates an environment where researchers can explore brain function more effectively.
Modeling Techniques
Modeling techniques are foundational to computational neuroscience. By constructing detailed simulations of neural networks based on known physiological data, researchers can predict how neurons interact under various conditions. A key characteristic of these techniques is the ability to replicate real-world brain functions in a controlled environment.
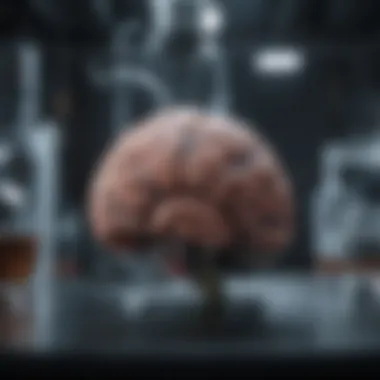
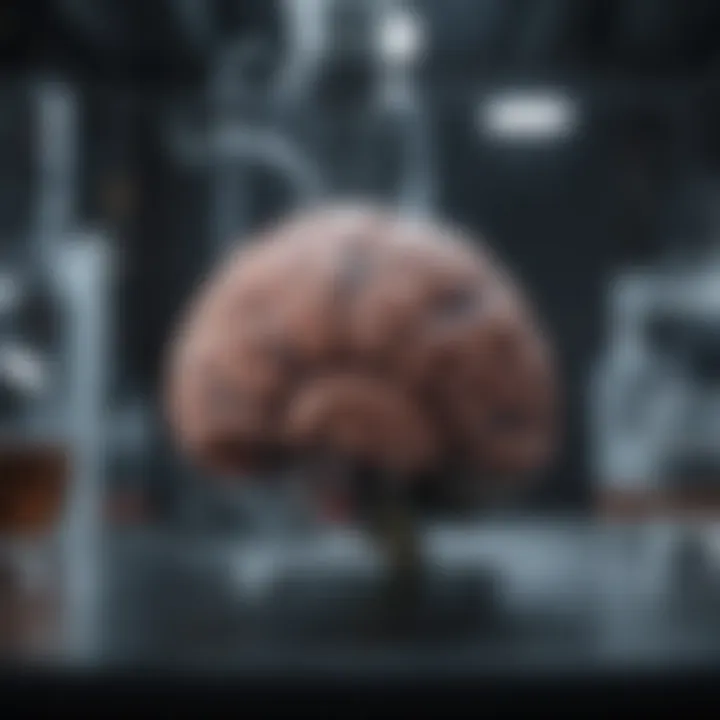
One major advantage of modeling techniques lies in their adaptability to different research questions. They can simulate both normal cognitive conditions and pathologies, making them a popular choice among neuroscientists. However, these models depend heavily on the accuracy of the input data. If the data is incomplete or inaccurate, the model's predictions can be misleading, which is a critical drawback.
Simulation Studies
Simulation studies go hand-in-hand with modeling techniques in providing insight into brain behavior. They involve creating approximations of brain processes to explore hypothetical scenarios. This approach is crucial because it allows testing of theories without the need to conduct complex and often ethically challenging experiments on live subjects.
The unique feature of simulation studies is their capability of running multiple scenarios through the same model, providing rich datasets for analysis. However, one must consider that simulation outcomes need rigorous validation against actual experimental data to ensure credibility.
In summary, current trends in brain lab protocols showcase how continuing advancements in neuroimaging and computational neuroscience are reshaping research methodologies. Each of these innovations provides a platform for enhanced data analysis and insightful understanding of brain functions, which ultimately drives the field of neuroscience forward.
Interdisciplinary Collaboration
Interdisciplinary collaboration stands as a cornerstone in brain lab protocols, not just a buzzword tossed around coffee breaks at conferences. Effective brain research mandates the input of diverse fields, embodying methodologies and perspectives that enhance outcomes. By intertwining the expertise from various domains, researchers can tackle complex questions and uncover insights that might slip through the cracks in siloed approaches.
Fields Related to Brain Research
Psychology
Psychology plays a pivotal role in understanding the human mind and behavior, adding depth to brain lab studies. The unique characteristic of psychology lies in its focus on mental processes and emotional responses. This emphasis becomes crucial when designing experiments that probe into cognitive functions or emotional responses related to brain activity.
In terms of contributions to research, psychology sheds light on how the brain influences behaviors and maintains mental health. For instance, cognitive-behavioral principles can guide the development of experimental tasks aimed at understanding decision-making processes in relation to brain functions.
One significant advantage here is the ability of psychology to integrate qualitative data, something that often gets lost in purely quantitative studies. However, a disadvantage could be the subjective nature of some psychological approaches, potentially introducing biases.
Engineering
Engineering, often overlooked amidst discussions of brain research, offers robust tools and methodologies that are essential. From developing neuroimaging devices to creating neural interfaces, the contributions from engineering are underpinned by innovation and precision. Key characteristics of engineering within this context include its rigorous methodology and its focus on practical application.
For example, in designing brain-machine interfaces, engineers must intimately understand not just the hardware, but also the neurological systems involved. This field directly contributes to making groundbreaking advances, such as enabling those with limited mobility to communicate through thought-driven technologies. However, one must also consider the complexity of the systems engineered, which can sometimes lead to unforeseen challenges during integration with biological systems.
Benefits of Cross-disciplinary Approaches
Cross-disciplinary approaches in brain research manifest numerous advantages. These include:
- Broader Perspectives: Incorporates diverse methodologies and understandings, enriching the research landscape.
- Increased Innovation: Combining different expertise can spark unique ideas that can push the boundaries of current knowledge.
- Richer Data: Integrating qualitative insights from psychology with the quantitative rigor of engineering provides a more comprehensive approach to investigating brain functions.
"In science, to pursue a deeper understanding, collaboration is no longer an option; it is a necessity."
Nevertheless, challenges also linger, such as communication barriers between professionals of differing backgrounds or aligning objectives towards a common goal. If these hurdles can be navigated thoughtfully, the rewards gleaned from interdisciplinary collaboration in brain research are truly invaluable.
Challenges in Brain Lab Protocols
The landscape of brain research is ever-evolving, and while remarkable strides have been made, a multitude of challenges persist. An in-depth understanding of these hurdles is crucial, as they can significantly impact the outcomes of research. Addressing these challenges not only guides the refinement of existing protocols but also catalyzes the development of innovative solutions conducive to progress in neuroscience.
Technical Difficulties
Technical difficulties in brain lab protocols can present a variety of obstacles, ranging from equipment malfunctions to procedural missteps. For instance, consider when a functional MRI machine fails mid-experiment. Not only does this halt the entire process, but it also leads to wasted resources and time, creating stress for researchers. Frustrations multiply when dealing with the complexities of specialized equipment, such as electrophysiology rigs or advanced neuroimaging systems.
Moreover, the continuous evolution of technology means researchers must stay updated with the latest advancements. This constant need for education and adaptation can strain both time and finances. Despite these challenges, organizations often tackle them by investing in meticulous training and maintenance schedules, emphasizing the importance of preventative measures.
Scientific Reproducibility
In the realm of neuroscience, reproducibility stands as a cornerstone of scientific integrity. If a study's findings can't be reliably reproduced, the research could potentially lose its significance and value. Several elements contribute to reproduction issues that permeate brain lab protocols.
Factors Affecting Reproducibility
One primary factor influencing reproducibility is variability in sample sourcing. When brain tissues or animal models are not derived under standardized conditions, discrepancies can arise in experimentation. For example, two laboratories adopting slightly different methods to handle and prepare mouse brain samples may yield vastly different neural responses. This inconsistency leads to a lack of reliability in representing the studied phenomenon.
Additionally, subtle distinctions in environmental factors—such as temperature, humidity, and even the inclination of floor tiles—can alter data. Recognizing these elements as crucial hurdles is foundational for enhancing reproducibility, ultimately driving towards more dependable scientific outcomes.
Frameworks for Improvement
In combating the issue of scientific reproducibility, frameworks for improvement offer structured pathways. One prominent example is the adoption of standardized protocols across institutions. This approach encourages uniformity in methodologies, thus enabling more coherent results when research is compared across labs.
Moreover, the implementation of rigorous peer review processes can serve as a safeguard to reinforce methodological integrity. Such frameworks prioritize transparency and meticulous documentation, allowing researchers to trace their steps back and identify potential errors in their procedures.
The benefits of these frameworks are noteworthy. Not only do they promote adherence to best practices within the neuroscience community, but they also foster collaborative efforts across research institutions. However, these frameworks may pose challenges as well, generating hesitance among researchers who are accustomed to their independent methodologies.
"Scientific progress is built on the foundations of reproducibility and reliability; addressing these challenges head-on can pave the way for innovative breakthroughs in brain research."
In closing, acknowledging and addressing challenges in brain lab protocols is paramount. From technical setbacks to reproducibility concerns, these hurdles highlight the necessity of ongoing education, training, and collaboration in the field. Through these concerted efforts, the journey of neuroscience continues to push the boundaries of understanding the human brain.
Future Directions
The realm of brain lab protocols is always evolving, and understanding where it is headed is pivotal for anyone in the neuroscience field. These future directions aren't merely projections but essential pathways that could redefine research methodologies. Exploring these emerging areas offers various benefits, such as improving research efficiency, enhancing the validity of results, and fostering rich interdisciplinary dialogue. Ultimately, these advancements can lead to a more nuanced understanding of brain functions and neurological disorders.
Emerging Areas of Research
Neuroscience and AI
Integrating Artificial Intelligence in neuroscience is increasingly becoming a hot topic. The application of machine learning in analyzing vast swathes of neurological data has opened up new vistas. The most notable characteristic here is AI's ability to process information at lightning speed, helping orient researchers toward significant patterns that might otherwise go unnoticed. This synergy serves as a beneficial choice in the realm of brain lab protocols because it augments traditional methodologies with robust analytical capabilities.
One unique feature of merging neuroscience with AI is the potential for predictive modeling. By training AI systems on data sets derived from brain imaging, researchers can predict outcomes in various experiments. However, it comes with its own set of challenges too—like ensuring the training data's quality and managing the ethical implications surrounding AI use. Nevertheless, the advantages overshadow the disadvantages when considering how AI propels neuroscience forward, making it a subject worth exploring in this article.
Brain-Machine Interfaces
Another exciting area is the development of brain-machine interfaces (BMIs). These systems offer a fascinating glimpse into direct communication pathways between the brain and external devices, which has profound implications for research and rehabilitation. A key characteristic of BMIs is their ability to translate neural activities into commands that machines can execute. This capability makes BMIs an invaluable choice as they provide real-time data critical for understanding brain functions.
The unique feature of BMIs is their potential for enabling control of prosthetic limbs or communication devices by thought alone. This has opened up new therapeutic avenues, especially for individuals with motor disabilities. However, the technology is still in its infancy and presents challenges such as signal noise and user variability. Despite these issues, their promise in advancing protocols makes them a crucial aspect of brain research moving forward.
Potential for New Protocols
The future is ripe with possibilities for developing new protocols tailored to meet the intricate demands of modern neuroscience. As various technologies converge and interdisciplinary collaboration deepens, the protocols will likely evolve into more specialized and efficient formats. By examining novel trajectories and integrating them into existing frameworks, researchers can supplement their understanding, enabling them to tackle intricate neurological questions more effectively.
Ultimately, these future directions hold the key to unlocking new frontiers in brain research. A deeper grasp of these emerging areas will significantly inform the foundational protocols we adhere to today, setting the stage for innovations that could reshape the landscape of neuroscience for years to come.