CRISPR and Genetic Engineering: A Detailed Overview
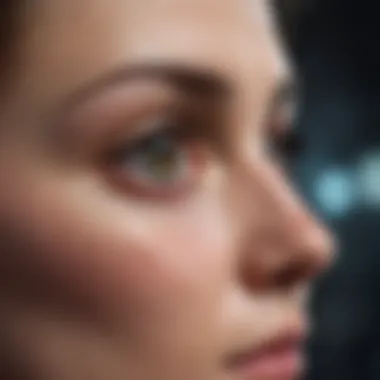
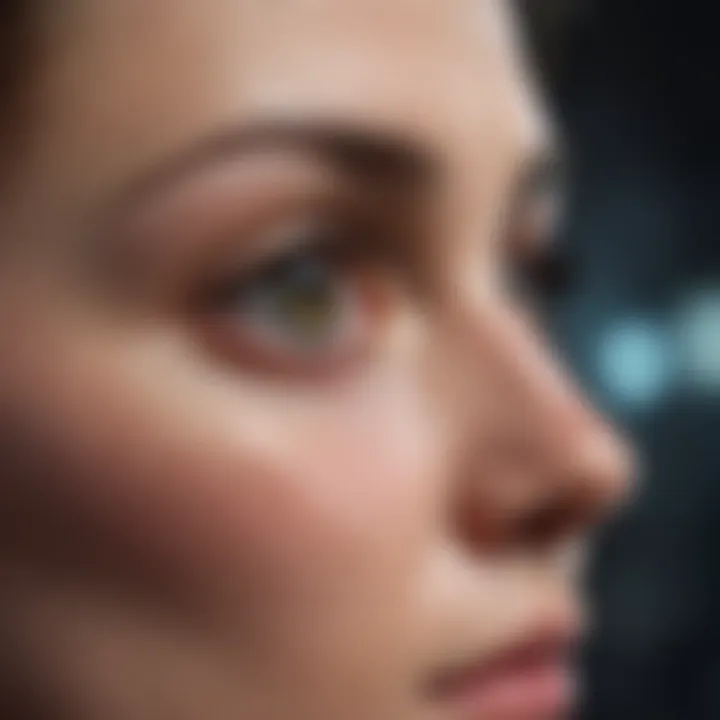
Intro
The advent of CRISPR technology marks a pivotal moment in the field of genetic engineering. This powerful tool allows scientists to edit genes like never before. Initially derived from a bacterial defense mechanism, CRISPR has rapidly evolved into a versatile instrument for researchers. Its precision and efficiency have opened new avenues for exploration in genetics, agricultural biotechnology, medicine, and more.
Understanding CRISPR entails not just the mechanics of how it operates, but also its implications for society and ethics. This article aims to unpack these complexities, providing insights into both the potential benefits and the challenges presented by gene editing technologies. As CRISPR technology continues to advance, discussions surrounding its applications and consequences become increasingly critical.
Researchers, students, and professionals alike are encouraged to engage with this topic, as it is not only at the forefront of scientific discovery but also touches on crucial ethical and regulatory considerations. In this examination, we will delve into the mechanisms behind CRISPR, the various applications of this technology, and the intricate web of legal and ethical frameworks that surround it.
Ultimately, the objective is to provide a comprehensive understanding of CRISPR and its role in shaping the future of genetic engineering, enriching the ongoing discourse on science and society.
Foreword to CRISPR
The exploration of CRISPR technology marks a significant milestone in the realm of genetic engineering. Understanding its foundation allows researchers and academics to appreciate its transformative potential. CRISPR, which stands for Clustered Regularly Interspaced Short Palindromic Repeats, represents not just a tool, but a paradigm shift in how we approach genomics.
Understanding CRISPR Technology
CRISPR technology functions as a precise and relatively simple means of editing genetic material. At its core, it mimics bacterial immune systems used to combat viral infections. Key components, such as CRISPR RNA and Cas proteins, allow this system to target specific DNA sequences with precision. This capability revolutionizes fields like medicine and agriculture.
The advancements afforded by CRISPR can lead to significant breakthroughs. For instance, gene editing through CRISPR allows for the development of therapies that could eliminate genetic disorders. Additionally, agricultural applications promise crops that can withstand environmental challenges and pests.
The simplicity of the CRISPR mechanism enables its accessibility. This means that not only well-funded laboratories but also smaller institutions can engage in impactful research. This democratization enhances innovation across diverse disciplines.
Historical Context of CRISPR Development
The path to CRISPR technology began in the early 2000s. Scientists first identified the unique genetic sequences within bacterial DNA. These sequences were recorded and studied, leading to the recognition of CRISPR's role in adaptive immunity.
In 2012, groundbreaking research emerged when Jennifer Doudna and Emmanuelle Charpentier demonstrated that CRISPR could be harnessed for targeted genome editing in higher organisms. This pivotal moment ignited significant interest across various scientific fields.
Following its discovery, the scientific community rapidly began experimenting with CRISPR. Different applications evolved, illustrating the technology’s broad scope. Research on genetic disorders, agriculture improvements, and even the potential for bioengineering were all influenced by the newfound understanding of CRISPR. This historical trajectory underscores the significance of CRISPR in modern science, shaping the ongoing discourse around genetic engineering.
"The advent of CRISPR technology has redefined the boundaries of genetic research. Its implications extend far beyond the lab, influencing ethical, social, and regulatory landscapes."
In summary, the introduction to CRISPR encapsulates both the scientific merit and societal implications of this groundbreaking technology. It sets the stage for understanding complex mechanisms, diverse applications, and the challenges that ensue in the pursuit of genetic innovations.
Mechanisms of CRISPR
The mechanisms of CRISPR underpin its revolutionary potential in genetic engineering. Understanding these mechanisms is essential for grasping how CRISPR can accurately edit genes. The interplay between various components like CRISPR RNA, tracrRNA, and Cas proteins allows for a sophisticated method of targeting and altering DNA. Thus, these mechanisms form the foundation upon which numerous applications in medicine, agriculture, and environmental science rely.
Components of CRISPR-Cas Systems
CRISPR RNA (crRNA)
CRISPR RNA, known as crRNA, plays a pivotal role in the CRISPR-Cas system. Its main function is to guide the Cas proteins to the target DNA sequence. crRNA is characterized by its unique ability to recognize specific DNA sequences through complementary base pairing. This specificity is a key advantage, as it ensures precise editing of the genome. In this article, crRNA stands out as a vital component due to its efficiency in directing DNA manipulation, making it indispensable for targeted gene editing.
Trans-activating RNA (tracrRNA)
Trans-activating RNA, or tracrRNA, works closely with crRNA to enhance the functionality of the CRISPR system. TracrRNA's defining characteristic is its capacity to bind with crRNA, forming a complex that can effectively guide Cas proteins to specific DNA sites. This pairing is beneficial because it stabilizes the crRNA and aids in the formation of the RNA-protein complex necessary for gene editing. The unique feature of tracrRNA is its ability to facilitate the recruitment of Cas proteins, thus amplifying the precision of genetic changes.
Cas Proteins
Cas proteins, particularly Cas9, are central to the gene-editing process. These proteins are nucleases that execute the actual cuts in the DNA. A critical characteristic of Cas proteins is their ability to create double-strand breaks at targeted sites, which is essential for gene modification. The selection of Cas proteins, especially Cas9, is popular due to its robust capability and effectiveness in various organisms. Cas proteins also present some disadvantages, such as the potential for off-target effects, which requires careful consideration when designing gene-editing experiments.
How CRISPR Edits Genes
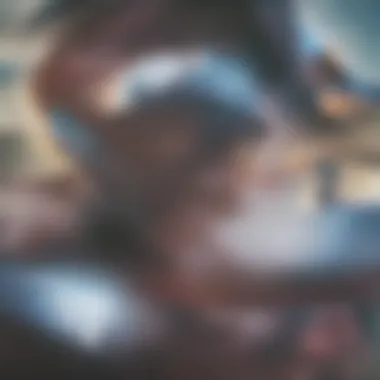
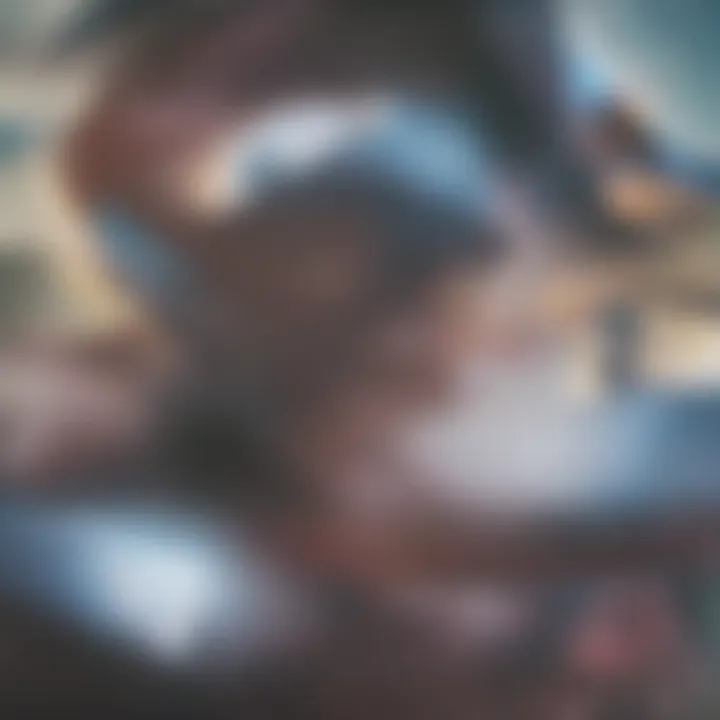
The gene-editing capabilities of CRISPR hinge upon three primary processes: targeting DNA, introducing double-strand breaks, and employing repair mechanisms. Each step contributes to the efficient modification of genetic material, ultimately enabling precise alterations.
Targeting DNA
Targeting DNA is the first step in the CRISPR gene-editing process. This involves the recognition of specific genetic sequences by the crRNA. The importance of this process lies in its precision, allowing for tailored modifications to be made. The distinctive feature of this targeting mechanism is its reliance on complementary base pairing, which provides a strong foundation for specific and accurate gene editing. The advantages of precise targeting are considerable, as they minimize unintended genetic alterations.
Introducing Double-Strand Breaks
Introducing double-strand breaks is a critical action carried out by Cas proteins following DNA targeting. This step is vital because it creates a gap in the DNA that can be repaired through various cellular mechanisms. The key aspect of this process is the strategic placement of breakpoints, which can dictate the type of genetic outcome achieved. By precisely directing breaks, researchers can induce the desired genetic changes, making this process a popular focus in genetic engineering.
Repair Mechanisms
After double-strand breaks occur, the cell's repair mechanisms come into play. These repair processes can lead to different outcomes, including non-homologous end joining or homology-directed repair. The significance of understanding these mechanisms lies in their impact on genomic stability and the nature of the resultant genetic modifications. A unique feature of these repair mechanisms is their inherent variability, which presents both opportunities and challenges. The advantages of harnessing these mechanisms include the ability to introduce precise edits, while the disadvantages may include unintended genetic consequences that arise from the repair process.
Applications of CRISPR in Genetic Engineering
The applications of CRISPR technology in genetic engineering are vast and critical. By enabling precise edits to be made within an organism's genetic material, CRISPR opens new avenues in various sectors, including healthcare, agriculture, and environmental science. This section will examine the importance of these applications and the implications they hold for the future of genetic manipulation.
Medical Applications
Gene Therapy
Gene therapy represents a significant breakthrough made possible by CRISPR technology. It involves the direct modification of genes to treat or prevent disease. By correcting dysfunctional genes, there is potential to tackle genetic disorders at their source rather than merely managing symptoms. The key characteristic of gene therapy lies in its specificity; CRISPR allows for targeted edits, which can minimize unintended consequences. However, while this approach is promising, factors such as delivery mechanisms and potential immune responses pose challenges that must be addressed.
Genetic Disease Research
Research into genetic diseases greatly benefits from CRISPR technologies. By utilizing this approach, scientists can create models of diseases that are more accurate. This enhances understanding and accelerates the discovery of treatments. The ability to manipulate genes accurately makes genetic disease research more effective and efficient. Yet, ethical concerns regarding the manipulation of human genes raise questions about the direction of such research and its implications for human health.
Immunotherapy Development
CRISPR plays a role in advancing immunotherapy, a treatment aimed at harnessing the body’s immune system to combat diseases, particularly cancer. By enabling precise modifications to immune cells, scientists can enhance the ability of these cells to identify and destroy cancerous cells. The distinct advantage of immunotherapy lies in its targeted approach, which often leads to fewer side effects compared to traditional treatments. Still, the possibility of off-target effects must be carefully managed.
Agricultural Innovations
Crops Resistant to Pests
Crops that can resist pests are crucial for sustainable agriculture. CRISPR enables the development of plant varieties that require fewer pesticides, which can lead to lower environmental impacts. This technique allows researchers to introduce resistance traits more efficiently than traditional breeding methods. However, consumer acceptance and potential impacts on biodiversity remain considerations that must be explored further.
Nutritional Enhancements
Nutritional enhancements in crops can be directly linked to CRISPR’s ability to edit genes associated with nutrient content. By improving vitamins or mineral levels, CRISPR-enhanced crops can contribute to better public health, especially in regions where malnutrition is prevalent. This application holds notable benefits, but it also encounters challenges regarding regulatory approval and public perception.
Mitigating Climate Change Effects
CRISPR's application in developing climate-resilient crops is particularly relevant in the current context of climate change. By editing the genetic makeup of plants, scientists aim to enhance traits such as drought tolerance or flood resistance. This potential to adapt agriculture to changing environmental conditions may ensure food security. However, concerns about the long-term effects of such modifications on ecosystems must be considered.
Environmental Applications
Conservation Genetics
Conservation genetics is another field where CRISPR has the potential to make a significant impact. By understanding the genetic diversity within endangered species, conservationists can use CRISPR to help maintain or enhance genetic variability. This aimed preservation can support species resilience against environmental changes. Nevertheless, the application raises ethical considerations regarding intervention in natural evolution.
Bioremediation Strategies
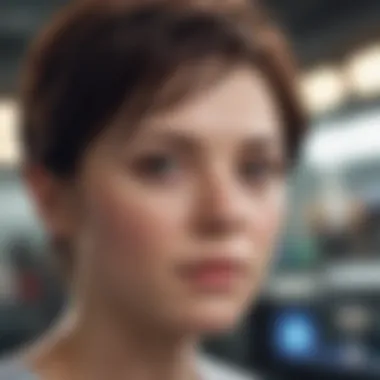
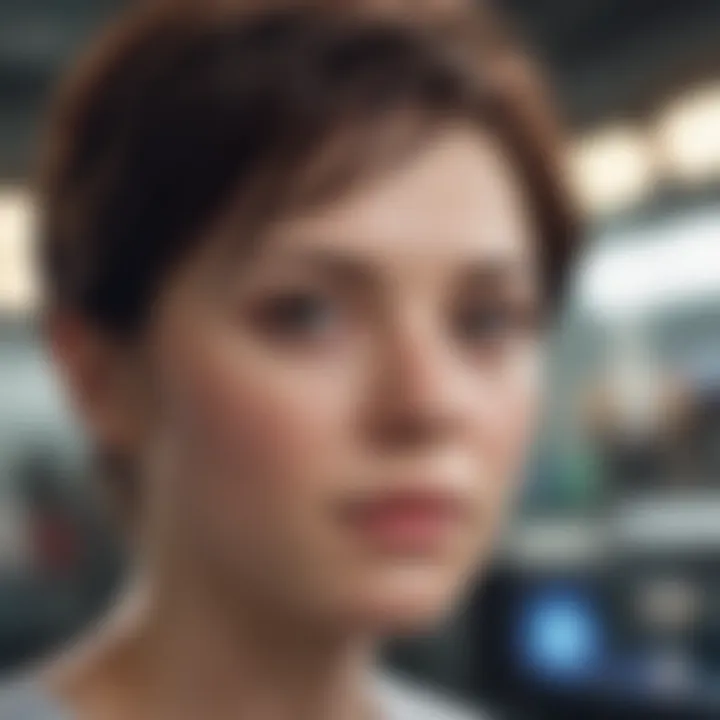
Bioremediation strategies involve the use of biological processes to address pollution. CRISPR can enhance the capabilities of microorganisms used in bioremediation by allowing the modification of genes that improve their ability to break down environmental pollutants. This specific aspect could lead to more efficient clean-up operations. Still, accountability and ecological implications of modified organisms in the wild are points of concern that require careful evaluation.
Overall, the applications of CRISPR in genetic engineering demonstrate potential transformative impacts across various sectors. The exploration of these areas must proceed cautiously, balancing innovation with ethical considerations.
Ethical Considerations in CRISPR Use
The use of CRISPR technology in genetic engineering raises significant ethical questions. These concerns are not merely academic but resonate deeply within the scientific community and wider society. It is essential to explore potential misuses, promote equity and accessibility, and address public perception and misinformation regarding gene editing.
Potential Misuse of Technology
Like any powerful tool, CRISPR can be misused. The potential for creating designer babies, for instance, stirs considerable ethical debate. This issue centers around genetic enhancement, which goes beyond treating genetic disorders to altering traits like intelligence or physical ability. There lies the risk of establishing a genetic divide, creating a society where genetic modification serves to exacerbate existing inequalities.
- Genetic Enhancement: Manipulating genes for non-medical enhancements could lead to unforeseen social consequences.
- Bioweapons: There is a tangible threat that CRISPR could be exploited to create harmful organisms or even engineered viruses.
- Illegal Human Cloning: Advances in gene editing might lead to illegal cloning practices for exploitative purposes.
Preventing misuse requires robust ethical guidelines, and continuing dialogue among scientists, ethicists, and policymakers.
Equity and Accessibility in Genetic Engineering
CRISPR has the potential to revolutionize medicine and agriculture, but the benefits must be evenly distributed. Currently, disparities exist in access to these technologies. Wealthier nations and individuals often benefit more than poorer ones. This raises questions over equity in genetic engineering.
- Access to Therapies: Gene therapies may remain inaccessible to many due to high costs.
- Research Investments: Institutions in affluent countries may receive more funding, sidelining researchers in developing regions.
- Global Inequality: Without equal access, CRISPR could worsen global health disparities.
Ensuring equitable access to CRISPR's advancements necessitates thoughtful policies and international cooperation to promote shared benefits.
Public Perception and Misinformation
Public understanding of CRISPR is crucial for its continued development and application. However, misinformation can shape perceptions negatively. Myths about genetic editing capabilities often circulate unchecked, fueling fear and misunderstanding.
- Media Influence: Sensationalized reporting can create unrealistic fears about CRISPR's potential.
- Lack of Education: Public education on genetic engineering needs to be improved to foster a more informed populace.
- Community Engagement: Involving communities in discussions about gene editing can mitigate fears and promote transparency.
Overall, addressing misinformation is vital for fostering public trust in genetic technologies.
Regulatory Frameworks Governing CRISPR
The regulatory frameworks governing CRISPR technology play a crucial role in ensuring its ethical application and effective oversight. These frameworks are essential for balancing scientific advancement with public safety and ethical considerations. With the rapid evolution of genetic engineering, there is a pressing need for well-defined guidelines and policies that can adapt to the fast-changing landscape of CRISPR research and applications.
Effective regulation can contribute significantly to various beneficial aspects of CRISPR utilization. For instance, it provides clear standards for researchers, helps prevent misuse, and ensures that advancements contribute positively to society. By addressing public concerns and uncertainties, regulatory measures can also foster trust and transparency. Furthermore, these frameworks can encourage innovation by offering a predictable environment for scientific inquiry.
National Guidelines and Policies
National guidelines and policies set the stage for CRISPR research within specific countries. Different nations approach genetic engineering with varying degrees of regulation. In the United States, the National Institutes of Health (NIH) and the Food and Drug Administration (FDA) play key roles in overseeing research involving genetic modifications, especially those aimed at human applications.
In contrast, countries such as Germany maintain stringent restrictions on genetic engineering, especially concerning germline modifications. This indicates a proactive approach to prevent potential ethical issues related to heritable changes.
- United States: The NIH provides oversight on funding for research involving genetic engineering, while the FDA evaluates therapies that engage CRISPR technology before they can be administered to patients.
- United Kingdom: The Human Fertilisation and Embryology Authority regulates all embryonic work involving CRISPR.
- Australia: The Gene Technology Regulator oversees the use of gene technology in both research and commercial applications, emphasizing compliance with the Gene Technology Act.
These frameworks not only shape research practices but also reflect the public sentiments and ethical discussions within the respective nations.
International Perspectives on Gene Editing
On an international scale, the perspectives on gene editing and CRISPR vary widely, influenced by cultural, ethical, and scientific factors. Organizations such as the World Health Organization (WHO) and the United Nations Educational, Scientific and Cultural Organization (UNESCO) contribute to establishing harmonized guidelines.
In some regions, there is a call for a moratorium on certain types of gene editing, particularly those that pertain to heritable modifications in humans. The
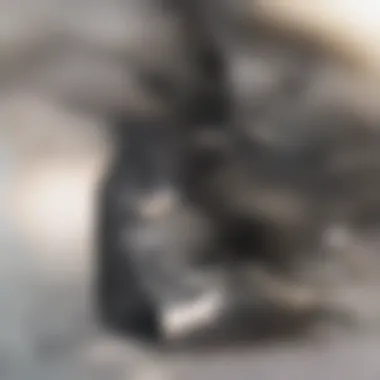
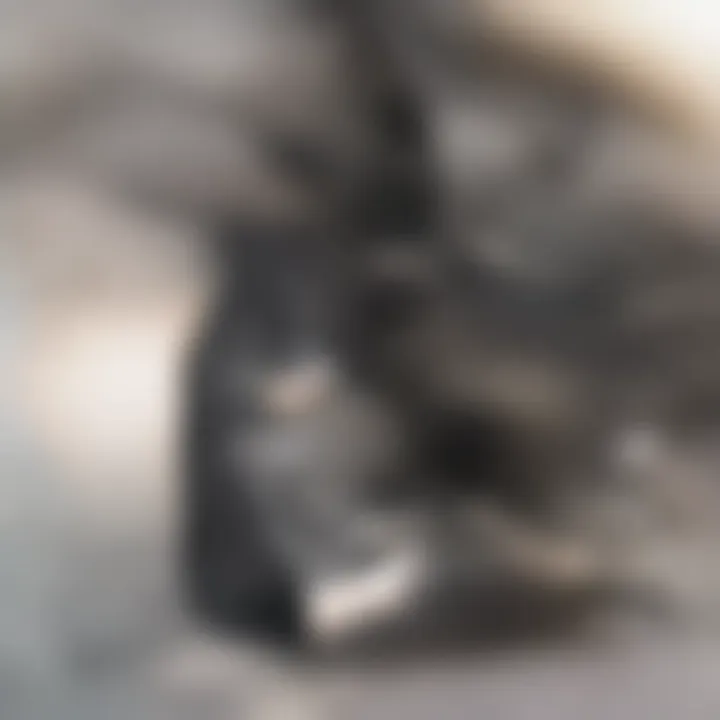
“Declaration of Toronto,” which emerged from international discussions, emphasizes the need for global dialogue on gene editing.
Conversely, countries at the forefront of biotechnology, like China and the United States, promote more lenient frameworks which facilitate agile research and development. These contradictory stances create challenges in international collaborations and may necessitate a harmonization of regulations to manage the global implications of CRISPR technology.
- Global Cooperation: Countries need to engage in discussions about shared ethical standards and responsibilities.
- Adaptability of Regulations: Regulatory frameworks must be dynamic to accommodate new discoveries and societal values as they evolve.
The incorporation of these diverse national and international perspectives is vital for establishing a cohesive framework that addresses safety, ethicality, and societal acceptance of CRISPR innovations.
Challenges in CRISPR Implementation
The advancement of CRISPR technology carries enormous potential but also presents unique challenges. Recognizing these challenges is crucial, as they can impact research scope, clinical applications, and public acceptance. In this article, we will delve into two significant challenges in CRISPR implementation: off-target effects and delivery mechanisms. These issues not only shape the future of genetic engineering but also determine how readily this powerful tool can be integrated into a variety of fields.
Off-Target Effects
One of the primary concerns with CRISPR technology is the occurrence of off-target effects. These unintended edits can lead to mutations in non-target genes, which may cause harmful consequences. Understanding the frequency and implications of off-target effects is essential for researchers. Studies have shown that while CRISPR is designed to target specific DNA sequences, it can also bind to similar, unintended sequences. This is particularly troubling in therapeutic applications, where precision is vital.
Here are some important aspects of off-target effects:
- Genomic Integrity: Off-target effects may compromise the integrity of an organism’s genome, leading to unforeseen consequences. This risk underscores the need for improving the specificity of CRISPR systems.
- Safety Concerns: The potential for unintended consequences raises significant safety concerns, particularly in human therapies. Regulatory agencies are cautious about approving treatments that demonstrate off-target risks.
- Refining Techniques: Ongoing research focuses on refining CRISPR techniques to enhance specificity. Innovative methods, such as high-fidelity Cas proteins, are being developed to minimize off-target hits, providing a more reliable tool for genetic editing.
"Off-target effects pose one of the greatest barriers to the widespread acceptance of CRISPR technology in medicine."
Delivery Mechanisms
To harness the power of CRISPR effectively, the selection of an appropriate delivery method is crucial. Causative agents, such as Cas9 and RNA components, need to be delivered to specific cells efficiently. However, achieving efficient and safe delivery remains a significant hurdle. The chosen method should ensure that adequate quantities of CRISPR components reach the desired target cells without unintended distribution.
Common delivery mechanisms include:
- Viral Vectors: These can introduce CRISPR components effectively but raise concerns about safety and immune responses.
- Lipid Nanoparticles: These are promising for encapsulating and delivering RNA, though their efficacy can vary.
- Microinjection: This technique is effective but not practical for a large number of cells, limiting its applications.
- Electroporation: This method can enhance delivery efficiency, but it may also damage the target cells.
The complexity of delivery factors highlights the necessity for ongoing research to identify methods that prioritize safety and effectiveness.
In summary, recognizing the challenges of off-target effects and delivery mechanisms is essential for advancing CRISPR technology. Addressing these obstacles will enhance our capacity to utilize CRISPR effectively and responsibly, opening new avenues for genetic research and therapeutic applications.
Future Perspectives
Exploring the future perspectives of CRISPR technology is crucial for understanding the evolving landscape of genetic engineering. As advancements continue, the implications for science, medicine, and society grow increasingly profound. This section focuses on several aspects that warrant attention. These include emerging technologies, the continuous refinement of methodologies, and the ethical concerns that will shape the application of CRISPR in the coming years.
Emerging Technologies in Gene Editing
The future of gene editing will likely be characterized by a wave of emerging technologies. Innovations are not just improving CRISPR itself but are also exploring complementary techniques. For instance, base editing, developed by researchers such as David Liu, offers a more precise method for making alterations to DNA sequences without introducing double-strand breaks. This method reduces the potential for off-target effects, a key concern in CRISPR applications.
Furthermore, prime editing is another groundbreaking advancement. Officially dubbed a "search-and-replace" technique, it allows for even greater precision in gene editing and could transform approaches in treating genetic disorders.
Techniques like these show promise for enhancing agricultural applications, improving resilience against climate change, and developing new therapies for complex diseases. These advancements usher in a new era where the limits of genetic engineering are being tested and expanded.
Impact of CRISPR on Future Research
The impact of CRISPR on future research is profound and multi-faceted. As researchers around the globe utilize CRISPR technology, it shapes various fields in unexpected ways. For example, in the realm of cancer research, CRISPR facilitates the development of targeted therapies that align treatments with individual patient profiles. This tailor-made approach enhances efficacy and reduces the side effects of traditional therapies, revolutionizing patient care.
In agricultural biotechnology, CRISPR's role will likely expand. Breeders can create crops that require fewer resources while yielding higher nutrition. This shift could help mitigate food scarcity issues predicted in coming decades.
Additionally, CRISPR is anticipated to expedite the pace of discovery in genetic engineering. By simplifying the gene editing process, researchers can pivot their focus to understanding gene functions and their interactions within biological systems. As a result, CRISPR is becoming a central tool in diverse research agendas, hinting at a future where understanding and manipulating genetic material becomes commonplace.
"The versatility of CRISPR will continue to open new doors in research previously thought closed."
In summary, the future perspectives of CRISPR technology extend beyond mere applications. As emerging technologies develop, they foster an environment ripe for innovation. The continuing democratization of gene editing will contribute to global health advancements and sustainable bioengineering solutions.
The specific benefits of these advancements emphasize the need for thoughtful and informed integration into society, encouraging responsible development and application of genetic tools.