Exploring Plastic Chemistry: Innovations and Insights
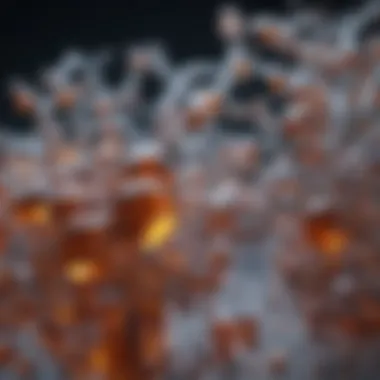
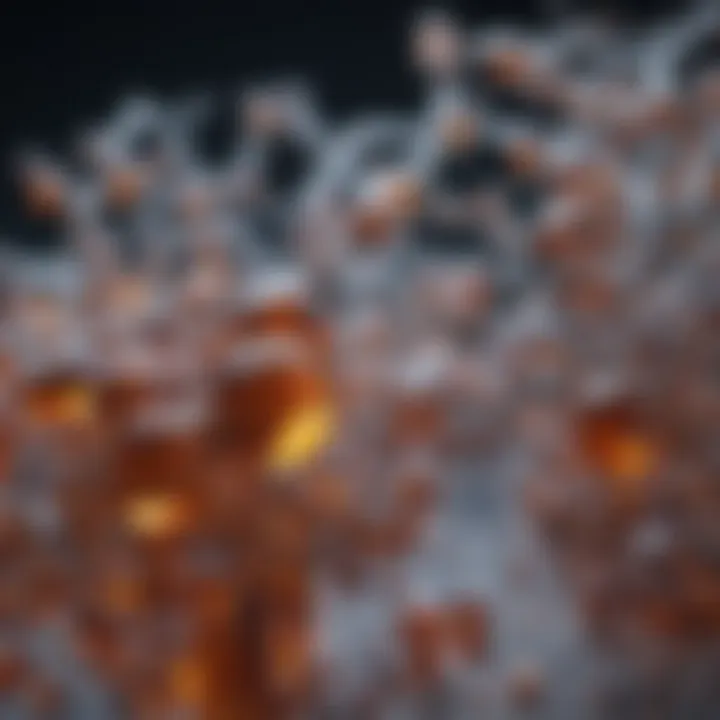
Intro
Plastic chemistry encompasses a fascinating interplay of various scientific principles, materials, and techniques that come together to shape our modern world. From the bags we toss aside to the intricate components in our latest gadgets, plastics have woven themselves into the fabric of everyday life. Although their convenience and functionality are undeniable, the environmental consequences of their production and disposal are beginning to cast a shadow on their many benefits.
In this piece, we aim to navigate the intricate pathways of plastic chemistry. We’ll dive into the foundational elements that govern polymer science, explore the different types of polymers, and examine the methods employed in their synthesis. Moreover, we will take a look at the environmental challenges posed by conventional plastics and highlight innovative strides being made in sustainability, demonstrating how this industry is adapting to growing ecological concerns.
Prepare for a comprehensive analysis that not only unpacks complex chemical structures but also presents practical insights that may appeal to students, researchers, and industry professionals alike. As we peel back the layers of plastic chemistry, we aim to shed light on the future trajectory of this vital field, laying the groundwork for further research and innovation.
Intro to Plastic Chemistry
In recent decades, the evolution of plastics has come to dominate a significant portion of our daily lives, shaping industries from packaging to automotive engineering. Understanding plastic chemistry is essential not only for those involved in the creation and application of these materials but also for anyone interested in comprehending the broader implications of plastic usage in modern society.
Defining Plastic Chemistry
At its core, plastic chemistry is the study of polymers, which are large molecules formed by repetitive subunits known as monomers. These macromolecules can be tailored to exhibit a diverse range of properties, making them suitable for a multitude of applications. The significance of plastic chemistry lies in its capacity to innovate; the right chemical formulation can yield materials that are flexible, durable, resistant to different environmental factors, and can even be designed for biodegradability or recyclability. Each type of polymer presents unique characteristics, demands specific processing techniques, and often has its own set of uses in various industries.
The umbrella term of plastic chemistry encompasses various disciplines, including organic chemistry, materials science, and even environmental science. Thus, mastering the fundamentals equips students, researchers, and industry professionals with essential tools for tackling some of today’s pressing challenges, such as plastic waste and sustainability.
Historical Development
The journey of plastic chemistry began in the early 19th century. One of the initial breakthroughs came with the invention of bakelite in 1907 by Leo Baekeland, which was heralded as the first synthetic plastic. This historical milestone did not just mark a new phase in material science but also paved the way for future innovations in polymer chemistry. It's fascinating how over the years, the focus shifted from the materials themselves to the implications of their usage and disposal, mirroring societal values and environmental awareness.
The mid-20th century ushered in an era of commercial prosperity for plastics, with an explosion in manufacturing and consumer goods. Innovations such as polyethylene and polypropylene became household names, leading to a plastic boom. However, as societal reliance on these materials grew, so did the concerns about their environmental impact.
Today, we dwell in a time marked by a duality: an appreciation for the versatility of plastics and an urgent call for sustainable practices. Understanding the historical context of plastic chemistry not only illuminates the incredible innovations that have occurred but also emphasizes the need for responsible production and consumption in light of contemporary challenges.
"The quest for sustainable materials is not merely an option; it’s a necessary evolution of our relationship with the environment."
In summary, the field of plastic chemistry stands at a crossroads, with the foundational knowledge and its historical developments serving as a backdrop against which future innovations and sustainable practices must unfold. Addressing these challenges will require ingenuity, technical proficiency, and an unwavering commitment to ethical considerations.
Fundamental Concepts of Polymers
Understanding the fundamental concepts of polymers is crucial to appreciating the entire landscape of plastic chemistry. These principles provide a foundation for comprehending how various types of plastics are made, their behaviors, and their applications. Likewise, recognizing the significance of polymer structure and properties can help in the quest for sustainable alternatives to conventional plastics, which have raised significant environmental concerns.
Polymer Structure
As we dive deeper into polymer structure, it becomes clear that the very makeup of a polymer governs its overall functionality. At the simplest level, polymers are long chains made up of repeated units called monomers. These monomers combine through a process called polymerization to form intricate structures that determine the polymer's characteristics.
Monomers vs. Polymers
Monomers, the building blocks of polymers, are small, low-molecular-weight molecules. Each monomer has specific properties that influence the behaviors of the resulting polymer. One could say that the choice of monomer is akin to selecting ingredients for a recipe; the final dish heavily depends on what you start with. The key characteristic of monomers is that they are reactive and can form covalent bonds with one another. This unique feature allows for a versatility that is highly beneficial in plastic chemistry.
On the flip side, once they form polymers, their characteristics dramatically change. A common disadvantage to note is that while polymers may exhibit desirable durability, they often lack biodegradability. Thus, understanding the transition from monomers to polymers and the interplay of their properties has a substantial impact on various applications in plastics today.
Linear, Branched, and Cross-Linked Structures
Within the realm of polymer structure, linear, branched, and cross-linked formations stand out as critical types. Linear polymers are formed when monomer units join in a straight chain, which often results in materials that can be melted and reshaped, such as polyethylene. Branched structures, on the other hand, consist of side chains extending from the main linear backbone. This leads to more complex properties, enhancing flexibility or crystallinity depending on the way the branching occurs.
Cross-linked polymers represent a whole different ball game. These structures have interlinked chains that create a network of connections. The increased bonding leads to materials that are more rigid and resistant to temperature changes, making them ideal for applications in car parts or kitchenware. However, a disadvantage here is that once set, they can’t be reshaped or recycled easily. Understanding the distinctions among these structures enables one to harness the unique advantages they provide in various applications and innovations within polymer science.
Polymer Properties
Polymers are not just defined by their structure but also by their properties. Knowing the physical and chemical properties of polymers helps in predicting their behavior under different conditions, facilitating practical applications.
Physical Properties
The physical properties of polymers, such as temperature resistance, tensile strength, and flexibility, play a pivotal role in determining their applications. For instance, the ability of a polymer to withstand environmental stresses can be the difference between a successful material in automotive parts versus one suitable for food packaging. A key characteristic of physical properties is that they are affected by the polymer's structure; for example, a tightly packed crystalline arrangement exhibits greater durability than an amorphous structure.
However, just like a double-edged sword, a unique feature of these properties is their susceptibility to thermal and mechanical stresses. While certain polymers may offer high strength, they might also embrittle under extreme conditions. Thus, understanding the balance of these traits is crucial when selecting materials for specific uses.
Chemical Properties
Chemical properties determine how a polymer will interact with its environment. For instance, certain polymers can be resistant to chemicals, making them suitable for containers that store reactive materials. The characteristics of chemical properties tend to be significant since they influence how a polymer reacts to heat, light, and various chemicals. A beneficial aspect of these properties is that properly engineered polymers can be designed to degrade under specific conditions, which plays into the growing hope for sustainable practices in plastic manufacturing.
However, their unique feature also encompasses vulnerabilities; some polymers can deteriorate when exposed to the elements, including UV light and moisture. Understanding these aspects is paramount, especially in a time when the longevity and environmental impact of materials are under scrutiny.
In summary, grasping the fundamental concepts of polymers— from their structural makeup to their essential properties—leads us to recognize their broader implications in the field of plastic chemistry. This knowledge serves as a stepping stone towards embracing innovations, particularly in sustainability that can help mitigate environmental impacts.
Types of Plastics
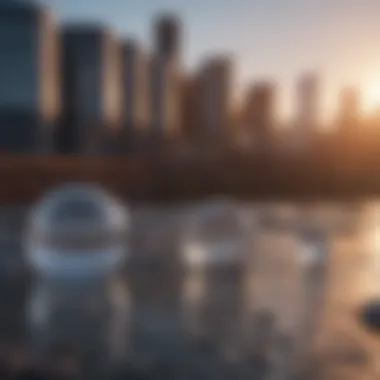
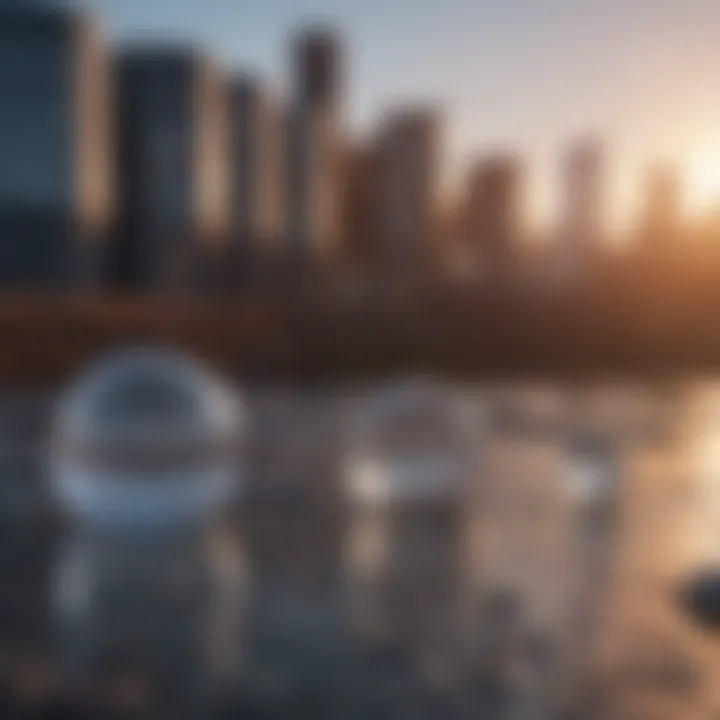
Understanding the different types of plastics is essential in the field of plastic chemistry. This section takes a closer look at three major categories: thermoplastics, thermosets, and elastomers. Each of these types has distinct characteristics and applications that impact various industries from manufacturing to consumer goods. Knowing the types of plastics not only helps in selecting the right material for specific applications, but it also provides insight into the environmental considerations and innovations associated with each type.
Thermoplastics
Thermoplastics are a family of plastics that become soft when heated and hard when cooled. This reversible process allows them to be reshaped multiple times without undergoing significant chemical changes. Common examples include polyethylene, polystyrene, and polyvinyl chloride (PVC). The versatility of thermoplastics stems from their ability to be molded and remolded, making them highly popular in packaging, automotive parts, and consumer products.
A key benefit of thermoplastics is their ease of recycling. Many of them can be reprocessed, which is an attractive quality in today’s environmentally conscious world. This reusability allows companies to reduce waste while also lowering costs associated with raw materials.
However, there are considerations as well. Thermoplastics can be sensitive to heat and UV radiation, which may lead to degradation over time. Modifications and additives often come into play to enhance their durability. From a practical viewpoint, understanding these nuances can lead to better design decisions in product development.
Thermosets
In contrast to thermoplastics, thermosets undergo a chemical change when heated, resulting in a hardened, cross-linked structure that cannot be remolded. Common thermosets include epoxy resins and polyurethanes. This characteristic gives thermosets exceptional strength and thermal stability, making them ideal for applications in aerospace, electronics, and automotive components.
One major advantage of thermosets is their resistance to deformation under heat and load, which can make them more suitable for demanding environments. They also provide excellent electrical insulation properties, making them valuable in the electronics industry.
However, their inability to be reshaped poses challenges in recycling. Once cured, thermosets are rarely recoverable, raising concerns over long-term sustainability. As the industry trends towards greener practices, the challenge lies in developing new ways to recycle or upcycle these important materials.
Elastomers
Elastomers are perhaps the most flexible of the plastic types, known for their elasticity – they can stretch and return to their original shape. This property makes them particularly useful in applications such as rubber bands, seals, and flexible tubing. Common examples are natural rubber and silicone.
The major advantage of elastomers lies in their ability to absorb energy and resist cracking or breaking under stress. These characteristics are crucial in applications where flexibility and durability are needed, like in automotive tires or seals for household appliances.
Nevertheless, elastomers can face issues with degradation from environmental factors such as ozone, heat, and oils. To combat this, stabilizers and fillers are often incorporated to improve longevity. Understanding these factors allows for better materials selection, which is essential when creating products designed for long-term use.
"The choice of the right plastic type can significantly impact efficiency, sustainability, and product performance."
Synthesis of Plastics
The synthesis of plastics represents a foundational pillar in the discipline of plastic chemistry, pivotal for transforming raw materials into versatile product forms employed across multiple sectors. Understanding this process not only sheds light on the transformative journey of base chemicals into everyday materials but also unveils the potential avenues for novel applications and innovations. The sophisticated interplay between various synthesis methods and the resultant properties of plastics greatly influences their performance, sustainability, and environmental footprint.
Polymerization Processes
Polymerization processes stand as the core mechanisms in the creation of plastics. These are categorized primarily into two types: addition polymerization and condensation polymerization. Each method comes with unique characteristics and implications, affecting everything from the molecular structure of the product to its eventual utility in consumer goods and industrial applications.
Addition Polymerization
Addition polymerization is a process where monomers react to form a polymer without the byproducts of other molecules. Its key characteristic lies in the ability to chain together many identical monomers, resulting in high molecular weight polymers. This method has become a beneficial choice due to its relatively simple and effective mechanism, allowing for the production of a variety of plastics, such as polyethylene and polystyrene.
A unique feature of addition polymerization is that it often employs specific initiators or catalysts to kickstart the reaction, which can lead to controlled polymer growth. This is advantageous in the sense that scientists can tailor the polymer properties, leading to diverse applications across packaging, construction, and even consumer electronics. However, the downside to this method can be found in the need for careful optimization of conditions to prevent runaway reactions or unwanted side reactions that could alter the desired outcomes.
Condensation Polymerization
Contrastingly, condensation polymerization involves the joining of monomers with the simultaneous loss of small molecules, often water. This method is particularly prominent in the production of polyesters and polyamides, notably in the creation of materials used in textiles or engineering components.
The key characteristic of condensation polymerization is its capability to create complex architectures, allowing for a diversity of properties that can be fine-tuned during synthesis. The attraction of condensation polymerization lies in its relative flexibility in designing polymer structures with varying functionalities, enhancing their applicability in sophisticated domains like biomedical fields.
However, one must acknowledge the inherent challenges; the removal of byproducts can often complicate the manufacturing process. It necessitates careful management to ensure efficiency and reduce environmental impact, highlighting the importance of this technique within the broader narrative of sustainable practices in plastic production.
Innovative Synthesis Techniques
As we shift focus to innovative synthesis techniques, it becomes clear that new methodologies are reshaping the landscape of plastic chemistry, fostering sustainability and efficiency in production.
Green Chemistry Approaches
Green chemistry approaches in polymer synthesis emphasize minimizing environmental harm and maximizing the use of renewable resources. Such strategies promote the use of non-toxic substances and energy-efficient processes, making them a beneficial choice in the pursuit of sustainable practices. By designing polymerization steps that either reuse waste materials or rely on renewable feedstocks, we can significantly reduce the carbon footprint associated with traditional methods.
A significant advantage of this approach is that it encourages innovation in sourcing materials, which can improve the commercial viability of biodegradable plastics. However, these methods are not without their challenges; the initial development costs may be high, and the scalability of these processes can often be a hurdle.
Nanotechnology in Polymer Synthesis
Nanotechnology in polymer synthesis marks a contemporary frontier that holds immense potential. This involves manipulating materials at the molecular level, yielding polymers with enhanced properties not attainable through traditional methods. The key characteristic of nanotechnology lies in its ability to create materials that boast superior strength, reduced weight, and unique functional behaviors, making them an appealing option for a wide range of applications.
The unique feature here emphasizes precision in design, where nanoparticles can be integrated to improve functionalities, such as conductivity in electronic applications. While the advantages are striking, this method presents challenges in terms of safety and environmental impact assessment, making regulations necessary as this technology advances.
"Innovations in synthesis not only redefine the possibilities of plastic production, but also illuminate pathways towards sustainable practices that benefit both industry and the planet."
In summary, the synthesis of plastics serves as a crucial part of plastic chemistry, bridging foundational knowledge with innovative practices that hold promise for the future. Each method, from polymerization to cutting-edge techniques, offers insights into how plastics can be designed to meet both current demands and future challenges.
Applications of Plastic Materials
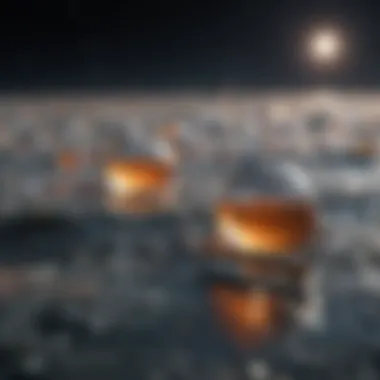
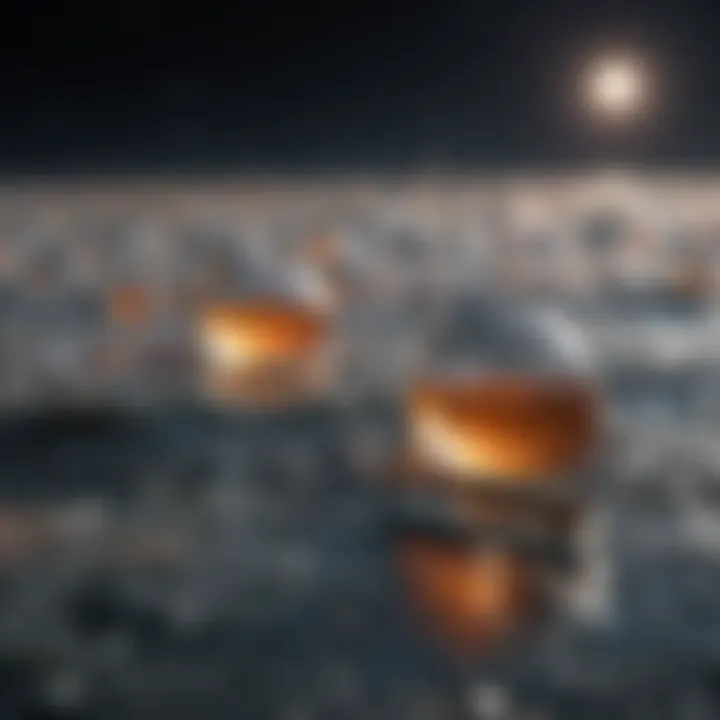
Understanding the applications of plastic materials is key to grasping the role they play in modern society. Plastics aren’t just mere artifacts; they are woven into the very fabric of multiple industries, yielding myriad benefits while also facing scrutiny regarding their environmental impact. The relevance of their applications extends beyond convenient usage; it harbors critical economic implications and shapes sustainable practices.
Industrial Applications
Packaging Industry
The packaging industry serves as a prime example of how plastic materials significantly contribute to efficiency and sustainability in daily commerce. Utilizing plastics for packaging offers a lightweight and strong protective layer around products, allowing for safer and more economical transportation over long distances. This thin yet durable material reduces overall waste as it often requires fewer resources compared to alternatives like glass or metal. One of the most notable aspects is its adaptability, allowing for various shapes and sizes, which can be tailored to an extensive range of products—from food to pharmaceuticals.
Among the key characteristics that make plastic a popular choice in this segment is its cost-effectiveness. By keeping production costs lower, manufacturers can pass on savings to consumers. This hardly makes plastics ideal but does raise questions about balancing convenience with environmental responsibility. An ongoing challenge is finding eco-friendlier options in packaging materials that can replace conventional plastics while ensuring the same level of efficiency.
Unique features, like biodegradable plastic containers, are now emerging. They present substantial advantages, such as reducing waste and promoting sustainable disposal practices. However, the challenge remains that not all biodegradable materials decompose effectively in natural environments, leading to claims of 'greenwashing.'
Automotive Engineering
In automotive engineering, plastics play a pivotal role in transforming vehicle design and functionality. From dashboards to bumpers, plastics provide lightweight solutions that enhance fuel efficiency by reducing overall vehicle weight. This not only benefits manufacturers in terms of production but also promotes environmental stewardship as efficient vehicles tend to exhibit lower carbon footprints.
A defining characteristic of plastics in this field is their versatility. Different polymer blends can mimic the properties of metals while offering design flexibility that is hard to achieve with harder materials. This trend allows automobile manufacturers to adopt more innovative shapes, contributing to both aesthetics and aerodynamics.
Nevertheless, challenges persist. While the lightweight nature of plastics reduces emissions during vehicle operation, issues of durability and recyclability pose threats. Automotive plastics are often single-use in their applications, leading to waste at the end of a vehicle's life cycle. Therefore, the transition to more sustainable materials, such as engineered bioplastics, must be prioritized.
Consumer Goods
Household Items
Plastic materials dominate the household items market due to their cost-effectiveness, sturdiness, and low production costs. Products ranging from containers to cleaning supplies are often made from durable plastics that withstand daily wear and tear, attracting consumers seeking longevity and practicality in their purchases.
An interesting feature of household plastic items is their customizability. Different polymers can be combined to design everything from kitchenware to home décor. These items can be produced in vibrant colors and various shapes, making them visually appealing for consumers.
However, popularity does not come without its downsides. The concerns surrounding plastic waste and the health implications of certain chemicals—such as BPA found in some food containers—necessitate deeper investigation into safer alternatives. Balancing functionality with safety is paramount for both manufacturers and consumers alike.
Electronics
The electronics sector showcases another layer of plastic application, with several components requiring insulation and protection. Plastics are extensively used in smartphones, computers, and other devices due to their excellent insulating properties and capacity to be shaped into intricate designs.
A key advantage of using plastics in electronics is weight reduction. Lightweight devices are not only favored by consumers but are also easier to transport and handle, adding to their value proposition. Furthermore, plastics tend to resist corrosion and moisture, which is crucial in improving the lifespan of electrical components.
On the flip side, the proliferation of electronics has led to a significant rise in electronic waste. Many plastic parts are non-biodegradable, complicating recycling efforts. Thus, the industry is exploring ways to integrate more sustainable practices, such as redesigning products for easier disassembly and promoting recycling programs.
"The diverse applications of plastics reveal the complex interplay between innovation and responsibility that industries must navigate in the face of growing sustainability concerns."
Overall, the applications of plastic materials underscore their undeniable utility, yet they also highlight the pressing need for innovation in sustainability. "Plastics are here to stay, but how we use them matters more than ever."
By understanding these facets, research and improvements can align towards sustainable alternatives without sacrificing the benefits that have made plastics a mainstay in various fields.
Environmental Impact of Plastics
The environmental impact of plastics is an ever-pressing concern that cannot be overlooked in the discussion of plastic chemistry. While plastics have revolutionized various industries and enhanced the functionality of countless products, their long-lasting effects on ecosystems and human health raise critical questions about sustainability and responsibility.
Plastic Waste Challenges
One of the most significant hurdles in managing plastic waste lies in its sheer persistence in the environment. Unlike organic materials that biodegrade, most traditional plastics take hundreds of years to decompose. This longevity leads to accumulation in landfills and the environment, creating mountains of waste that choke landscapes and waterways. The impact is particularly evident in urban areas where proper disposal methods are often lacking. Millions of tons of plastic waste find their way into oceans each year, with disastrous consequences for marine life.
"Every piece of plastic that has ever been created still exists in some form today."
Moreover, the economic burden is not to be underestimated. Municipalities spend vast sums on waste management, struggling to keep up with increasing volumes of discarded plastic. The costs associated with cleaning up plastic debris from natural habitats or urban settings only add to this financial strain. Solutions such as recycling are often hampered by issues like contamination and the economic viability of processing recycled materials.
- Key Challenges:
- High rate of plastic production and consumption
- Ineffectiveness of current recycling methods
- Insufficient waste management infrastructure
Microplastics and Ecosystems
The emergence of microplastics has further complicated the narrative surrounding plastic pollution. These tiny plastic particles, often measuring less than five millimeters, originate from larger plastic debris that breaks down through processes like sun exposure, wave action, and mechanical stress. Microplastics have been discovered in even the most remote regions of the planet, from pristine Arctic ice to the depths of the ocean.
Their presence poses significant risks to ecosystems. Microplastics are often ingested by marine organisms, entering the food chain and potentially impacting human health as well. Aquatic life, including fish and filter feeders, mistake these particles for food. The implications are dire: toxins from plastics can accumulate in the bodies of these creatures, leading to bioaccumulation and biomagnification up the food chain. This raises immediate concern for communities that rely on fishing, as contaminated fish can impact food safety and public health.
- Effects on Ecosystems:
- Alteration of habitats and biodiversity
- Disruption of food chains
- Potential human health risks through seafood consumption
Sustainable Alternatives and Innovations
As discussions around plastic usage evolve, the spotlight turns to sustainable alternatives and innovations in this field. This section is a deep dive into the paths being paved to address the environmental hurdles plastics present. Sustainable alternatives not only tackle the growing plastic waste problem but also embody a shift toward a more responsible and circular economy. Exploring the benefits of biodegradable plastics and advanced recycling technologies, we can appreciate their roles in shaping a greener future.
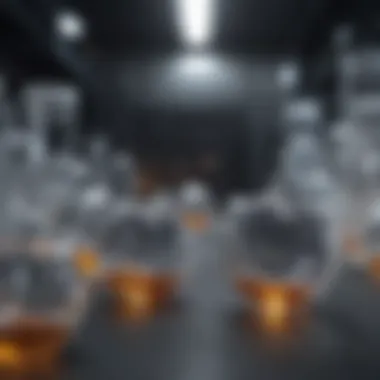
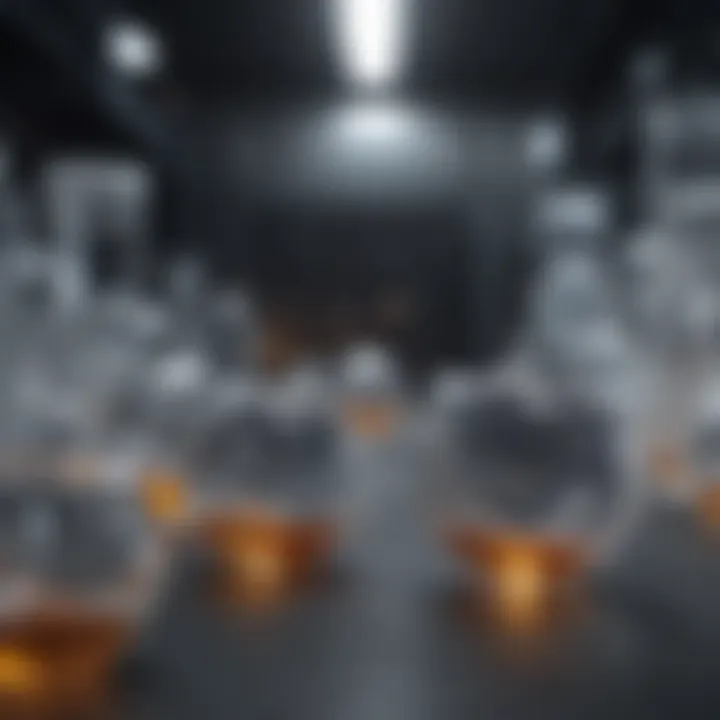
Biodegradable Plastics
Sourcing Materials
When it comes to sourcing materials for biodegradable plastics, the focus shifts toward renewable resources. This choice significantly influences the environmental footprint of plastic production. Corn starch, sugarcane, and other plant-based materials are often used. What sets these materials apart is their ability to break down naturally, turning into non-toxic components over time. By selecting natural sources, manufacturers can reduce reliance on fossil fuels, making it a more popular option in discussions of sustainability.
However, this avenue isn’t without its challenges. Cultivating crops for bioplastics can compete with food production, raising ethical questions about land usage and resource allocation. Ultimately, the benefit lies in developing a cycle that contributes positively to the economy while lowering pollution levels accompanying traditional plastics.
Commercial Viability
The commercial viability of biodegradable plastics is increasingly gaining traction. It’s not just about making eco-friendly choices; businesses want tangible returns. That said, innovations in production processes and increasing consumer demand render these materials appealing. One of the key characteristics of biodegradable plastics is their potential to command higher market prices compared to conventional plastics due to growing environmental awareness.
Despite these advantages, a unique feature of commercial biodegradable plastics is how they are perceived in terms of performance. Many of these products may not match the durability of regular plastics, which may deter some companies from making the switch. Moreover, issues surrounding the proper disposal of these materials can complicate their marketability. But, as technology advances, and more consumers prioritize green options, this trend toward commercial viability is bound to expand further.
Recycling Technologies
Mechanical Recycling
Mechanical recycling is a pivotal player in the quest for sustainable plastic use. This method essentially involves the physical reprocessing of plastics into new products. The main advantage of mechanical recycling is its relative simplicity and cost-effectiveness. Plastics collected from various waste streams can be sorted, cleaned, and formed into pellets or flakes for reuse in new products.
However, one of the downsides is the degradation of polymer quality, which can limit how many times a plastic can be recycled while maintaining its properties. This compromise may lead manufacturers to shy away from mechanically recycled materials due to concerns about durability or performance. But with attention on innovations in processing technologies, it’s likely that enhanced mechanical recycling solutions will emerge in the future.
Chemical Recycling
On the other hand, chemical recycling represents another innovative approach capable of reshaping the recycling landscape. This process breaks down plastic polymers into their core monomeric building blocks, enabling the resurrection of high-quality plastic. One of the standout features of chemical recycling is its ability to process complex plastics that mechanical recycling struggles with.
Despite its benefits, the drawbacks are not negligible. The energy-intensive nature of chemical recycling can be a hurdle. It requires careful consideration of the environmental impact of such processing methods. Nonetheless, as the need for better recycling technologies grows, chemical recycling stands at the forefront as a potential game-changer in addressing plastic waste effectively.
"The shift towards sustainable capitalism is not just beneficial, but essential for future generations to come."
In summary, implementing sustainable alternatives and innovations such as biodegradable plastics and advanced recycling techniques presents an opportunity to move toward a circular plastic economy. These pathways not only promise a reduction in environmental impact but also challenge manufacturers and consumers alike to rethink their relationship with plastic. As we embrace both commercially viable strategies and eco-friendly materials, we can navigate the complexities of plastic use in a more conscientious manner.
Regulatory and Ethical Considerations
In today's world, the push towards responsible plastic use has gained considerable momentum. While plastics offer immense benefits, their production and disposal come with significant implications for both the environment and human health. Regulatory and ethical considerations serve as the framework to navigate these complexities. When discussing plastic chemistry, it is crucial to explore how regulations shape the industry and reflect our societal values, ensuring sustainable practices in the face of growing environmental concerns.
Global Regulations on Plastic Use
Globally, regulations surrounding plastic usage vary widely, reflecting local economic conditions, environmental priorities, and public health concerns. A few major frameworks include:
- The European Union Plastics Strategy: This initiative aims to make all plastic packaging recyclable by 2030. It also sets ambitious targets for reducing single-use plastics.
- The United States Environmental Protection Agency (EPA): While regulation in the U.S. can be more fragmented, the EPA plays a pivotal role by enforcing standards that prevent pollution resulting from plastic production and disposal.
- Asia-Pacific Initiatives: Countries like Japan have pioneered legislation that emphasizes plastic waste reduction, encouraging recycling and proper waste management.
Though regulations intend to address the pressing issues, they often lead to debates on enforcement, economic feasibility, and effectiveness. Therefore, involvement from both policymakers and communities is essential for creating frameworks that resonate with societal needs and environmental goals.
"The regulation of plastic use isn't just bureaucracy; it’s a necessity for paving a sustainable future for our children."
Ethics of Plastic Production
The ethics of plastic production encompasses a myriad of topics, from the sourcing of raw materials to labor practices in manufacturing plants. As awareness grows, several ethical considerations have come to the forefront:
- Sourcing Materials: The extraction of fossil fuels for plastics poses risks, including ecological damage and potential harm to local communities. Ethically sourced alternatives are being explored to mitigate these impacts.
- Labor Practices: There are ongoing concerns about working conditions in factories that produce plastic. Advocating for fair labor practices is essential to ensure that the industry's growth does not come at the cost of human dignity.
- Consumer Responsibility: As plastic usage remains prevalent, there's a call for consumers to make informed choices regarding the products they purchase. Understanding the lifecycle of plastics is part of this ethical framework.
In summary, addressing regulatory and ethical considerations empowers stakeholders to engage in meaningful discussions about sustainable practices in the plastic industry. By developing comprehensive policies and prioritizing ethical production methods, we can help ensure a future where plastic chemistry serves both humanity and the planet.
Future Trends in Plastic Chemistry
The field of plastic chemistry is on the brink of transformative changes, driven by innovations aimed at addressing both environmental crises and the growing demand for sustainable materials. This section delves into the future trends that are shaping the landscape of plastic chemistry, underscoring its significance in improving the ecological footprint of plastic use. As researchers and industries alike increasingly prioritize sustainability, understanding these trends becomes crucial for anyone involved in or studying this field.
Advancements in Polymer Science
In recent years, the evolution within polymer science has been staggering. Researchers are now exploring ways to enhance the characteristics of existing polymers and create entirely new types. One key area of advancement lies in the development of functionalized polymers that integrate bioactive elements. These polymers may be used in medical applications, such as drug delivery systems, where controlled release is necessary for therapeutic effectiveness.
Furthermore, the combination of biopolymer materials with traditional plastics is gaining traction. For instance, blending polylactic acid (PLA) with other thermoplastics can produce materials that retain desirable properties while offering better biodegradability. The challenge, however, lies in ensuring that these new materials maintain their practical functionalities without compromising performance.
In addition, there’s a growing interest in smart polymers, which respond dynamically to environmental stimuli. These include changes in temperature, pH, or light, offering promising applications in fields ranging from healthcare to environmental monitoring. As polymers evolve, they have the potential to become multifunctional materials, paving the way for innovations that could minimize waste and resource consumption.
The Role of Innovation in Sustainable Practices
Innovation isn't just a buzzword; it's the lifeblood of sustainability in the plastic industry. The integration of sustainable practices into plastic chemistry is becoming increasingly vital. One significant trend is the emergence of circular economy principles within plastic production. This approach emphasizes the continuous use of resources, as opposed to the traditional linear model of production and disposal.
Several companies are investing in advanced recycling technologies, which allow for the breakdown of plastics into their constituent monomers, enabling the creation of new plastic products without sacrificing quality. This method contrasts sharply with traditional recycling, often leading to inferior products and greater waste.
Another innovative area includes the development of bio-based plastics sourced from renewable materials such as cornstarch or sugarcane. These alternatives can significantly reduce reliance on petrochemicals, presenting a vital step towards a greener future for plastics. Furthermore, ongoing research into enzyme-based recycling methods shows promise in enhancing the efficiency of breaking down complex polymer structures, potentially revolutionizing the recycling process.
"Innovation isn't just about creating; it's about rethinking how we do things to preserve our planet for future generations."
These advancements demonstrate an essential shift in focus within the industry. Rather than merely producing plastics, companies are increasingly required to consider the entire lifecycle of their products, from origin to disposal. This holistic view fosters an environment where material sustainability is as important as material functionality.