An In-Depth Exploration of Quantum Physics
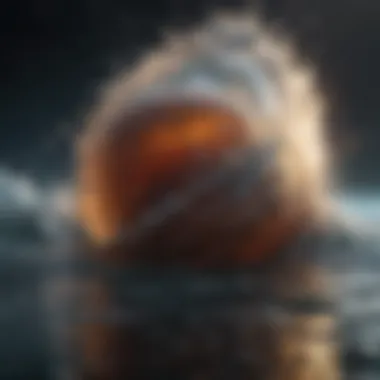
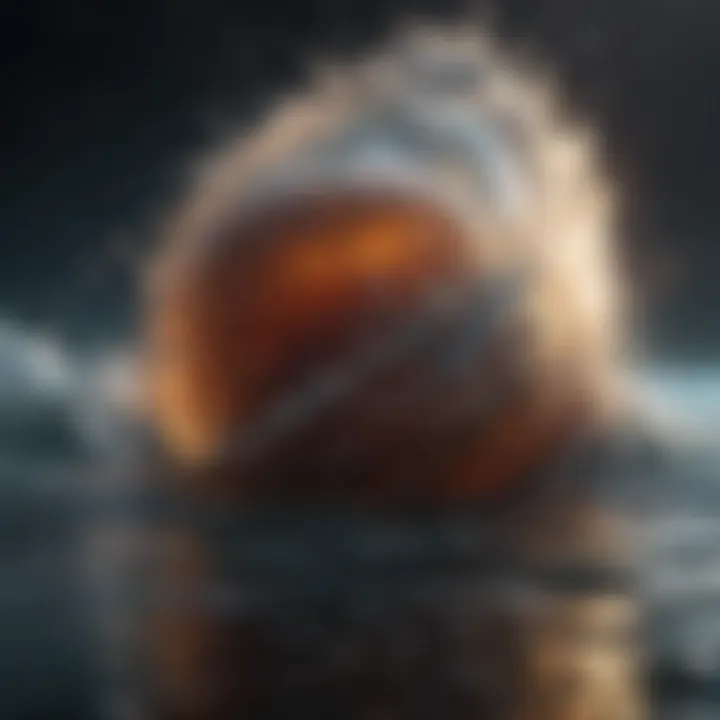
Intro
Quantum physics, a field of science that challenges our traditional understanding of nature, deals with the behavior of matter and energy at the smallest scales. This article sets out to unravel the complexities inherent in quantum physics, exploring the fundamental principles, key experiments, and profound implications of this branch of physics.
As researchers and academics delve deeper into this field, it is essential to grasp the key concepts such as wave-particle duality, uncertainty principle, and quantum entanglement. These concepts not only shape our understanding of the universe but also catalyze advancements in technology, influencing various industries. The interplay between theory and experimental validation creates a rich tapestry of inquiry, making quantum physics a pivotal area of study for today's scholars.
In this exploration, readers will find an organized narrative marked by clear headings, facilitating an insightful understanding of quantum physics. The article will thoroughly cover essential aspects of this subject, catering to varied inquiries, while remaining accessible to students, researchers, educators, and professionals.
Research Overview
Summary of Key Findings
Quantum physics indicates that particles behave like both waves and particles. This duality forms the basis of many phenomena observed in quantum mechanics. Another key finding is the uncertainty principle, formulated by Werner Heisenberg, which asserts that certain pairs of properties, like position and momentum, cannot be precisely measured at the same time. Furthermore, quantum entanglement reveals how particles become interconnected, allowing changes in one to affect another, regardless of the distance between them.
Significance of Research
The implications of quantum physics extend far beyond theoretical musings. Technologies such as quantum computing, quantum cryptography, and advanced imaging techniques are grounded in principles of quantum theory. This research informs not only the scientific community but also industries aiming to innovate and evolve. Understanding quantum physics is crucial for the development of future technologies, promising a new frontier in both science and technology.
"Quantum mechanics is not just a theory; it is a framework that has transformed our comprehension of reality."
Methodology
Research Design
The exploration of quantum physics is largely based on theoretical frameworks supported by experimental validation. Researchers design studies to test predictions derived from quantum theory, using different methodologies, such as controlled experiments and theoretical simulations.
Data Collection Methods
Data in quantum physics typically comes from experiments involving advanced technology like particle accelerators and quantum sensors. The observations from these experiments allow researchers to collect data about the behavior of quantum systems, hence confirming or refining existing theories.
Preamble to Quantum Physics
Quantum physics represents a fundamental shift in how we understand the universe. It delves into the behaviors of matter and energy at the atomic and subatomic levels. This exploration is essential for anyone looking to grasp modern physics and its implications in technology and theory.
One significant element of quantum physics is its challenge to classical physics' principles. Classical mechanics sufficed for macroscopic objects but fails at smaller scales. Thus, understanding quantum physics allows deeper insights into atomic interactions and phenomena that defy ordinary logic.
This section introduces key concepts that shape our modern understanding of technological advancements. From quantum computing to cryptography, the implications of quantum physics are broad and transformative. By laying a solid foundational knowledge, we can appreciate how quantum physics shapes numerous fields, providing a robust framework for further exploration in the article.
Definition of Quantum Physics
Quantum physics is the branch of physics that studies the smallest units of matter and energy. It describes how particles such as electrons and photons behave differently than larger objects in our everyday lives. Key principles include quantization, which suggests energy exists in discrete units, and wave-particle duality, where particles exhibit properties of both waves and mass.
Historical Background
The Plank Era
The early 20th century marked the beginning of what we now call the Plank era, named after Max Planck. He proposed that energy is not continuous but comes in small packets, or quanta. This breakthrough laid the groundwork for quantum theory. Planck's work was pivotal because it offered a new perspective on energy emission and absorption. One remarkable characteristic of this era was the birth of quantum theory, which changed how scientists approached physics. The unique feature of the Plank era is its focus on how small-scale events can lead to vast implications in physical laws.
The Development of Quantum Theory
As the 20th century progressed, several key developments in quantum theory emerged. Notable advancements were provided by the works of Albert Einstein and Niels Bohr. Einstein's explanation of the photoelectric effect showed that light has both wave-like and particle-like properties. The development of quantum theory was crucial because it bridged the gaps in classical physics. A unique aspect is its ability to provide predictions that are verified experimentally, highlighting its importance. However, the increase in complexity of quantum concepts often leads to misunderstandings in broader society.
Key Figures in Quantum Physics
Numerous prominent figures shaped the framework of quantum physics. For instance, Werner Heisenberg introduced the uncertainty principle, noting the limitations in measuring both position and momentum of particles simultaneously. Key figures contribute different perspectives to the common goal of understanding atomic behavior. These contributions are vital for discussing the evolution and relevance of quantum theories. Their various interpretations and findings underscore both the richness of exploration in this field and the eventual complexities that arise from varying viewpoints.
Importance of Quantum Physics
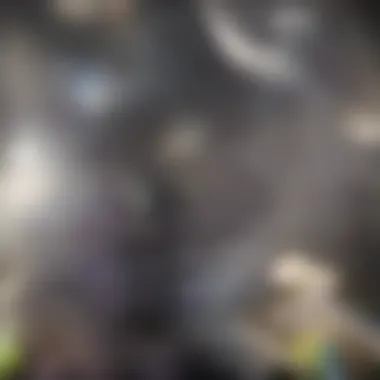
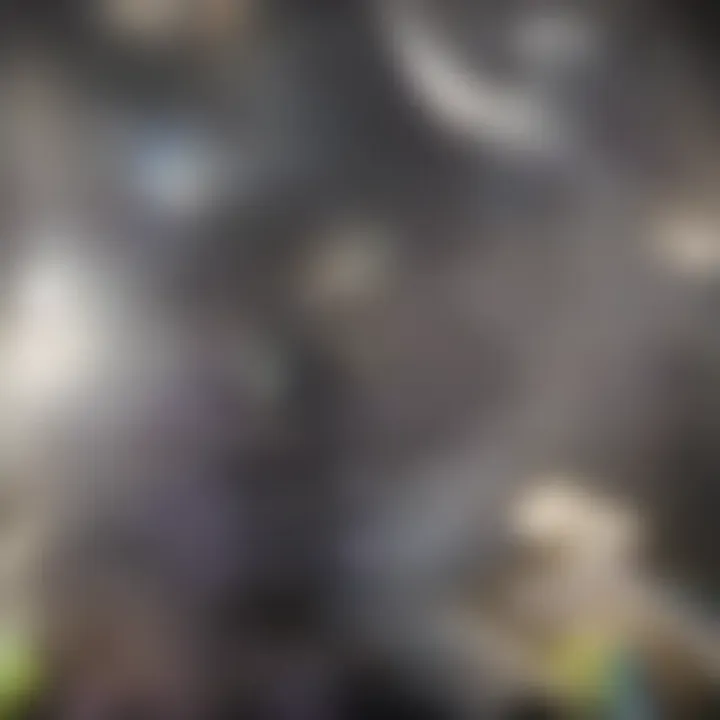
The importance of quantum physics cannot be overstated. It fundamentally changes our understanding of the universe. This field influences various domains including electronics, materials science, and biology. As technology advances, quantum principles are fundamental in designing new devices.
Moreover, quantum physics contributes to a deeper philosophical inquiry into the nature of reality and observation. The subtle interactions at the quantum level challenge our perceptions of determinism and causality. A profound realization of this field is its intersection with everyday life, and how it shapes modern technology.
"Quantum mechanics forces us to reconsider fundamental assumptions about the nature of reality."
Understanding quantum physics fosters better awareness of the technological developments stemming from this rich field. From creating more efficient solar panels to developing quantum computers, the applications are vast and significant.
Core Principles of Quantum Physics
The core principles of quantum physics serve as the foundation for understanding the behavior of matter and energy at the subatomic level. These principles are not merely theoretical constructs; they have profound implications for various fields, including technology, philosophy, and our worldview. Their significance lies in how they challenge classical physics, offering insights that are essential for advancements in modern science and technology.
Wave-Particle Duality
Wave-particle duality is one of the central themes of quantum physics. It proposes that every particle or quantum entity exhibits both wave and particle properties. This duality means that an object can behave like a particle in certain experiments and like a wave in others. The discovery of this characteristic emerged from experiments such as the double-slit experiment, where light can create interference patterns typical of waves, but also generate discrete packets of energy, photons, which behave like particles.
This concept is critical because it alters our understanding of the nature of light and matter. For researchers, it opens up a new path to understanding fundamental interactions. It also has consequences for technologies like lasers and semiconductors.
Quantum Uncertainty Principle
The quantum uncertainty principle, formulated by Werner Heisenberg, states that certain pairs of physical properties, like position and momentum, cannot both be precisely known at the same time. This intrinsic limitation challenges the classical idea that all properties can be measured with arbitrary precision.
This principle is important as it introduces a fundamental limit to what can be measured, representing a significant shift in our understanding of measurement in physics. It highlights the probabilistic nature of quantum mechanics, making it essential in quantum theory and applications such as quantum cryptography.
Quantum Superposition
Quantum superposition states that a quantum system can exist in multiple states at once until it is observed. This means, for example, that an electron can be in different energy levels simultaneously. Only when a measurement is made do we observe a definite state.
The concept of superposition is crucial for understanding quantum computing, where bits can be in a state of 0, 1, or both simultaneously. This allows quantum computers to perform complex calculations more efficiently than classical computers.
Quantum Entanglement
Definition and Explanation
Quantum entanglement is a phenomenon where particles become interconnected, such that the state of one particle instantly influences the state of the other, regardless of the distance separating them. This relationship persists even when the particles are light-years apart, suggesting a profound level of interconnectedness in the universe.
Entanglement is notable for its implications in quantum information science, including quantum computing and quantum cryptography. The mysterious nature of entangled particles raises questions about locality and the fundamental nature of reality itself.
Experimental Evidence
Experimental evidence for quantum entanglement has emerged through several key experiments, including those based on Bell's theorem. These experiments demonstrate that entangled particles do not adhere to classical physics’ expectations and instead exhibit correlations that cannot be explained by classical theories.
This characteristic of quantum entanglement has practical implications in developing quantum technologies, like quantum teleportation and secure communication. The experiments contribute to establishing entanglement as a robust phenomenon, cementing its place in our understanding of quantum mechanics.
The principles of quantum physics redefine our understanding of reality, pushing the limits of what we can quantify and perceive.
Fundamental Experiments in Quantum Physics
Fundamental experiments in quantum physics serve as the cornerstone for the entire field. They provide not only validation of theoretical frameworks, but also catalyze the evolution of technology based on quantum mechanics. Such experiments challenge classical intuitions about reality, demonstrating phenomena that starkly contrast with everyday experiences. Understanding these experiments deepens one’s grasp of the principles at play and showcases the boundary between the known and the mystery that continues to invite inquiry.
The importance of these experiments cannot be overstated. They reveal the nuances of quantum behavior, such as the duality of light and matter, and help elucidate abstract concepts. By studying these experiments, researchers can cultivate a clearer understanding of quantum mechanics, and apply this knowledge in various fields, including quantum computing, cryptography, and materials science.
Double-Slit Experiment
The Double-Slit Experiment is a pivotal demonstration in quantum physics that significantly underscores the wave-particle duality of light and matter. In this experiment, light is directed at a barrier with two parallel slits. When both slits are open, an interference pattern appears on the detection screen, indicative of wave-like behavior. However, when particles, such as electrons, are sent through one at a time, they also create an interference pattern over time, suggesting that they behave as waves.
The intrigue escalates when one considers the role of measurement in this experiment. When an observer tries to detect which slit a particle passes through, the interference pattern disappears, implying that the act of measurement collapses the particle’s wave function into a definite state. This phenomenon raises profound questions about the nature of reality and observation, challenging conventional concepts of existence.
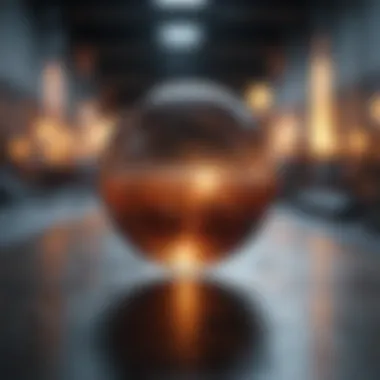
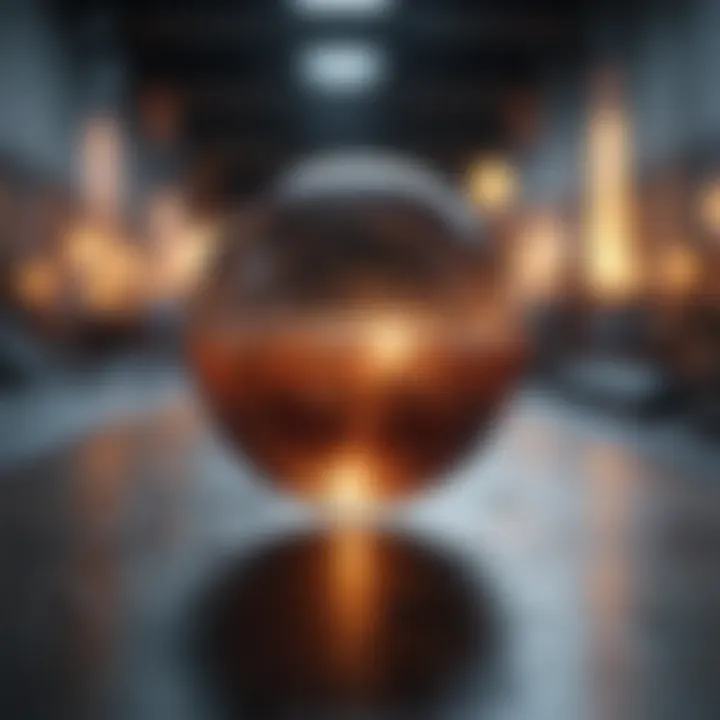
Photoelectric Effect
The Photoelectric Effect is another fundamental experiment that demonstrates the quantization of light and is critical in explaining how light interacts with matter. When light shines on a metal surface, electrons are ejected if the light's frequency exceeds a certain threshold. This observed phenomenon cannot be explained by classical wave theories but aligns with Albert Einstein’s interpretation of light as being made of packets of energy called photons.
The implications are vast; the photoelectric effect laid the groundwork for quantum mechanics and solidified the concept of energy quantization. It is also fundamental to various technologies, including solar panels and photo-sensors. The event of photons striking a material and causing the ejection of electrons has practical applications in photonics and energy conversion, emphasizing its significance.
Bell's Theorem and Experiments
Bell's Theorem envelops a series of powerful experiments that confront the perplexities of quantum entanglement and local realism. John Bell formulated this theorem to distinguish between the predictions of quantum mechanics and those rooted in classical ideas about independent particles. The core of Bell's theorem revolves around the correlations predicted for entangled particles, which demonstrate non-local interactions.
Experiments testing Bell's Theorem have provided critical evidence supporting the non-locality of quantum mechanics. They show that when two entangled particles are measured, the outcome of one particle instantly influences the other, regardless of the distance separating them. This phenomenon challenges classical intuitions about separability and locality in physics.
By exploring these pivotal experiments in quantum physics, one can grasp not only the mechanics of the quantum world but also the philosophical implications that arise from them. It encourages a profound inquiry into the nature of reality and our understanding of the universe.
Applications of Quantum Mechanics
Quantum mechanics has profound implications across various fields, driving innovation and technological advancements. It provides a framework for understanding phenomena that classical physics cannot explain. The applications of quantum mechanics are diverse, ranging from computing technology to secure communication. This section explores three prominent areas: Quantum Computing, Quantum Cryptography, and Quantum Teleportation.
Quantum Computing
Principles of Quantum Computing
Quantum computing relies on the principles of quantum mechanics, specifically superposition and entanglement. Unlike traditional computers, which use bits as the smallest unit of data, quantum computers use qubits. A qubit can exist in multiple states simultaneously due to superposition, enabling quantum computers to perform complex computations far more efficiently than classical counterparts.
One key characteristic of quantum computing is its ability to solve specific types of problems exponentially faster. For example, algorithms like Shor's algorithm can factor large numbers in polynomial time, which is a significant advancement in cryptography and computational efficiency. This unique feature makes quantum computing a promising choice in fields that require immense data processing and analysis.
However, it is essential to note the challenges associated with quantum computing, such as error rates and qubit coherence. These issues must be addressed for the technology to reach its full potential.
Challenges and Future Directions
The future of quantum computing comes with its challenges. One major aspect is the need for stable qubits. Maintaining their coherence is crucial for effective computation. Researchers are actively exploring new materials and architectural designs to enhance stability.
A notable characteristic of this field is the ongoing work to develop quantum error correction protocols. These protocols address the inherent errors in quantum computations and are a focus area for many research institutions.
Quantum Cryptography
Quantum cryptography employs principles of quantum mechanics to create secure communication channels. Unlike traditional methods, quantum cryptography leverages the uniqueness of quantum states to enhance security. Key protocols, like Quantum Key Distribution (QKD), ensure that any attempt at eavesdropping will be detectable.
The main benefit of quantum cryptography is its ability to guarantee the integrity and confidentiality of data. With increasing cyber threats, the importance of robust security measures cannot be overstated. Organizations and governments are exploring QKD as a feasible defense against potential attacks.
However, practical deployment presents challenges, such as the need for specialized equipment and infrastructure. As research progresses, the hope is that quantum cryptography will become more accessible and widely implemented.
Quantum Teleportation
Quantum teleportation refers to the transfer of quantum information from one location to another without the physical transport of the underlying particles. This intriguing concept relies on quantum entanglement, allowing two parties to share information instantaneously.
The principal application of quantum teleportation lies in quantum communication networks. By utilizing this technology, it is possible to achieve secure and efficient data transmission over long distances.
Nevertheless, quantum teleportation is still in experimental stages, and the practical implementation raises technical challenges. Scalability and the stability of quantum states during the teleportation process are critical issues researchers are addressing.
In conclsion, the applications of quantum mechanics are transforming technology and communication. While there are challenges that remain, the advancements made thus far offer promising pathways for future developments and innovations in quantum technologies.
Philosophical Implications of Quantum Physics
The intersection of philosophy and quantum physics invites profound questions regarding the nature of reality, observation, and the role of the observer in the measurement process. These implications extend beyond theoretical pursuits and stimulate discourse in ethics, epistemology, and the foundations of scientific inquiry. By exploring these philosophical implications, researchers and students can gain a nuanced appreciation of quantum physics. This section delves into interpretations of quantum mechanics such as the Copenhagen and Many-Worlds interpretations and discusses their respective impacts on our understanding of reality.
Interpretations of Quantum Mechanics
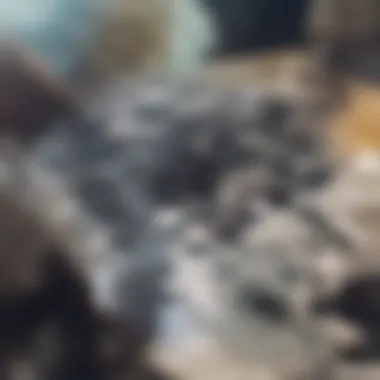
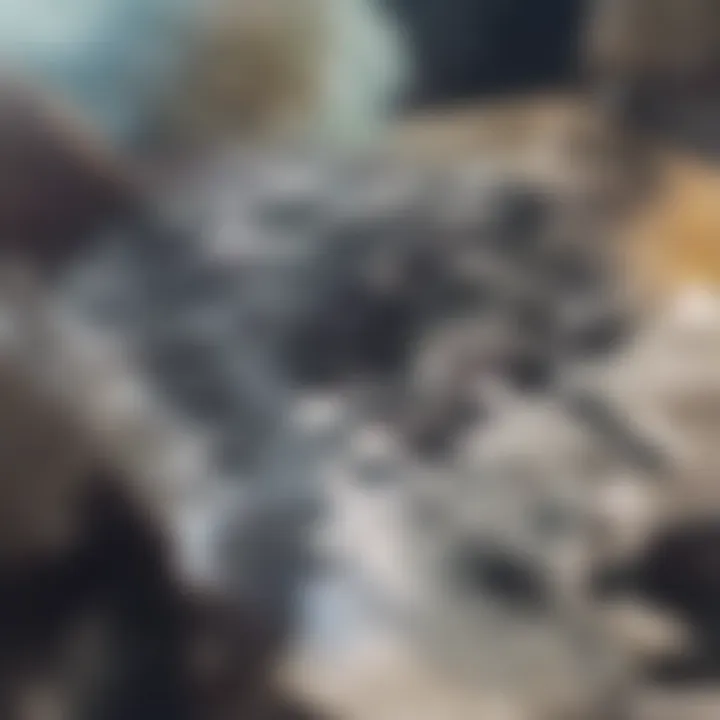
Copenhagen Interpretation
The Copenhagen Interpretation, developed primarily by Niels Bohr and Werner Heisenberg in the early 20th century, is a cornerstone in the philosophy of quantum physics. It posits that physical systems do not have definite properties until they are measured. This reliance on observation suggests a fundamentally probabilistic nature of particles. The key characteristic of this interpretation is the principle of wave function collapse, meaning that the act of measuring a quantum state causes it to adopt a specific value.
This interpretation is beneficial for students and researchers as it provides a clear framework for understanding how quantum measurements work. It simplifies complex phenomena into a more digestible form. However, its reliance on the observer raises philosophical concerns. Critics argue that it undermines objective reality. The unique feature of the Copenhagen Interpretation is its pragmatic approach, emphasizing experimental outcomes over theoretical constructs. While it has been widely accepted in the scientific community, its limitations prompt debates about reality's nature in quantum mechanics.
Many-Worlds Interpretation
In contrast to the Copenhagen Interpretation, the Many-Worlds Interpretation, proposed by Hugh Everett III in the 1950s, suggests that all possible outcomes of a quantum event actually occur. Every measurement leads to a branching of the universe into multiple, coexisting realities. This interpretation presents a radically different view, implying that an infinite number of parallel universes exist and that every quantum decision leads to a new dimension of reality.
The Many-Worlds Interpretation is appealing for its bold approach to resolving issues such as wave function collapse. It preserves the determinism of quantum mechanics while eliminating the observer's role in creating reality. However, its complexity presents challenges. The unique feature of this interpretation is its vastness, allowing for innumerable outcomes and potential realities. This characteristic can be both an advantage, offering deeper exploration into quantum phenomena, and a disadvantage, leading to difficulties in testing its predictions.
Impact on Reality and Observation
The philosophical implications of quantum physics fundamentally alter our understanding of reality and the role of observation. Traditional views in physics dictated that reality is objective and independent of human perception. Quantum physics provokes reconsideration of these assumptions, suggesting that the observer plays a critical role in shaping the observed reality.
"In quantum mechanics, the observer effect emphasizes that the observer's choices can influence outcomes."
This premise raises questions about the very nature of knowledge and existence. Are we mere participants in a reality shaped by our observations? Furthermore, the implications extend beyond physics and find relevance in various fields such as epistemology and metaphysics, fostering a rich ground for discussion in both science and philosophy.
In summary, the philosophical implications of quantum physics challenge conventional views of reality. They invite ongoing inquiry into how we understand existence and our place within it. By examining interpretations like the Copenhagen and Many-Worlds models, we can engage with the foundational questions of quantum theory, urging researchers and students to think critically about the nature of reality itself.
Contemporary Challenges and Research in Quantum Physics
The field of quantum physics is continuously evolving, presenting both opportunities and difficulties for researchers and practitioners. Addressing these contemporary challenges is vital not only for the advancement of quantum theory itself but also for the practical applications that stem from it.
Current Research Frontiers
Current research in quantum physics explores several frontiers. These include quantum computing, quantum communication, and the fundamental aspects of quantum mechanics. In quantum computing, researchers aim to develop algorithms and hardware that can leverage quantum bits, or qubits, to perform calculations at unparalleled speeds. Major companies like IBM and Google are making progress in this area, aiming to create reliable quantum computers.
The potential of quantum communication for secure data transmission is also a significant focus. Quantum key distribution is a promising method enabling two parties to exchange cryptographic keys securely, impervious to eavesdropping. This capability is beneficial for applications in finance and national security.
Another frontier is exploring the fundamental principles of quantum mechanics. Researchers are investigating how these principles can lead to new technologies. It involves not just theoretical studies but also practical experiments testing the limits and implications of quantum theory.
"Quantum mechanics is a beautifully intricate theory that yields results which often challenge our classical intuitions."
Unresolved Questions
Despite the progress, many unresolved questions persist in the field of quantum physics. One of the most pressing is the measurement problem, which pertains to how a quantum system transitions from multiple probabilities to a single outcome. This issue touches on the interpretations of quantum mechanics and raises philosophical questions about reality and observation.
Another unresolved question involves the true nature of quantum entanglement. While experiments have shown its existence, the underlying mechanism remains unclear. It is crucial to clarify whether entangled states imply instantaneous actions across distances or if they reveal a deeper connection in space-time.
Furthermore, how do quantum phenomena scale from microscopic to macroscopic worlds? Understanding this scaling issue could lead to breakthroughs in bridging quantum physics with classical physics.
In summary, contemporary challenges and research in quantum physics encompass a broad range of inquiries. These questions not only fuel academic research but also have practical implications for technology. The answers to these challenges may significantly alter our understanding of the universe and the development of future technologies.
Closure
The conclusion serves as a vital element in summarizing the main insights presented in this exploration of quantum physics. It not only encapsulates the core aspects of the discourse but also ties together the various sections discussed throughout the article. Understanding quantum physics is essential, particularly in today's world where technology relies heavily on quantum principles.
Summary of Key Points
This article has traversed numerous significant themes related to quantum physics, including:
- Core Principles: Wave-particle duality, the uncertainty principle, and quantum entanglement.
- Fundamental Experiments: Notable experiments such as the double-slit experiment and the photoelectric effect that illustrate these principles.
- Applications: The relevance of quantum computing, cryptography, and teleportation, showcasing how quantum theories are shaping modern technology.
- Philosophical Implications: Engaging interpretations and their effect on our understanding of reality.
- Contemporary Challenges: Ongoing research fronts and unresolved questions that continue to intrigue scientists and researchers.
Summarizing these elements highlights the intricate relationship between theoretical constructs and practical applications, emphasizing the framework that quantum physics provides for making sense of complex phenomena.
Future of Quantum Physics
The future of quantum physics holds massive potential for both technological advancement and conceptual understanding. Key points for consideration include:
- Technological Integration: As quantum computing progresses, we anticipate exceptional leaps in processing speed and capacity, impacting fields like artificial intelligence and data analysis.
- Research in Quantum Theory: Unsolved questions may lead to new theories or revisions in existing models, significantly altering our grasp of physical laws.
- Societal Implications: Improved quantum cryptography could lead to enhanced security in digital communications, reshaping the landscape of data protection.