Comprehensive Insights into Scientific Assays

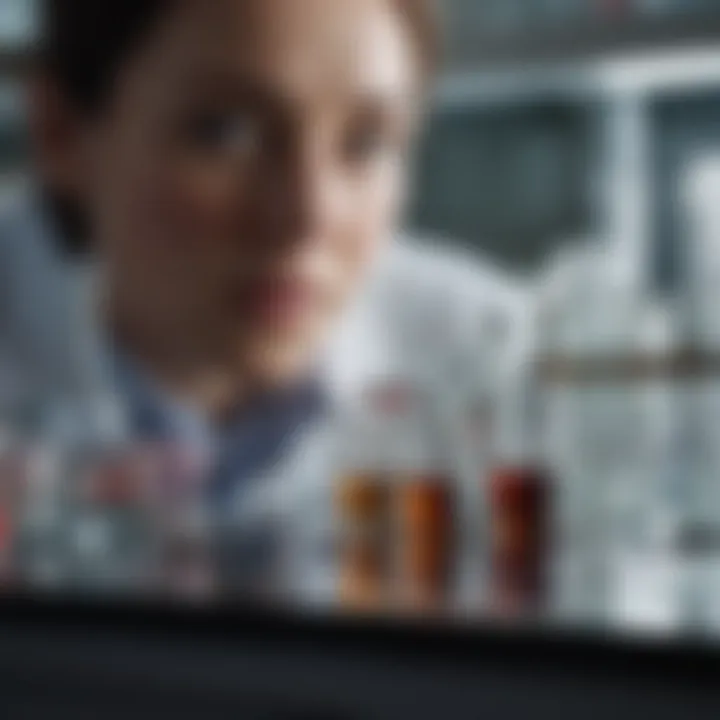
Intro
Scientific assays have anchored their importance in the vast realms of research and industry, serving as the bedrock of many discoveries and technological advancements. The word ‘assay’ might sometimes evoke images of complex laboratory procedures, hidden beneath layers of technical jargon, but at its heart, it’s a straightforward concept. An assay fundamentally measures the presence, amount, or activity of a substance. Whether in the pharmaceutical world, environmental science, or diagnostics, these methodologies make it possible to extract meaningful data from a seemingly chaotic array of physical and chemical interactions.
Over the years, these assays have evolved. Today’s assays can be seen as an intricate tapestry woven with threads of biology, chemistry, and physics. They don’t just help in identifying a compound but reveal the intricate stories behind how and why those compounds interact with one another.
As we embark on this exploration, it’s vital to recognize the historical background that shaped modern scientific assays. Understanding this will not only equip researchers with context but will also shed light on the significant milestones achieved throughout the years. Historical perspectives often provide insights into the evolution of methodologies and how they adapted in response to challenges and innovations in technology.
In the forthcoming sections, we will delve into the various classifications of assays, from biological to physical ones, and assess their respective roles in contemporary research. By unraveling the complexities of scientific assays, we aim to furnish students, researchers, educators, and professionals alike with a nuanced understanding of these crucial methodologies.
Intro to Scientific Assays
Scientific assays are integral to the framework of modern research, serving as the backbone for experimentation and analysis across various fields. They provide systematic ways to evaluate the characteristics or functions of substances, whether biological, chemical, or physical in nature. Recognizing their importance is essential for anyone engaged in scientific work, as these techniques not only enhance our understanding but also propel innovation.
Defining Scientific Assays
A scientific assay can be broadly defined as a procedure that quantifies the characteristics, presence, or concentration of a substance. These procedures can take many forms, depending on the context and the substances being measured. For instance, one common type is the biological assay, used to determine the effects of a drug on living organisms. In the pharmaceutical realm, assays might examine how a certain compound interacts with a receptor in the body. A chemical assay, on the other hand, might focus on identifying the concentration of a reagent in a reaction mixture.
The essence of an assay lies in its reliability and reproducibility. Each procedure must adhere to strict guidelines to ensure that results are valid and can be replicated by others. This raises the bar for scientific integrity and governs the way researchers communicate their findings. In simple terms, a well-structured assay is like a well-conducted orchestra, where every element plays its role precisely to produce meaningful results.
Importance in Research
The significance of scientific assays cannot be overstated. Here are several aspects highlighting their critical role in research:
- Facilitating Discoveries: Assays help researchers uncover new data and insights. For instance, when exploring drug efficacy, an assay can demonstrate not only if a drug works but also how well it performs compared to existing treatments.
- Promoting Standardization: In research, standardizing methods is crucial. Assays provide a wide-ranging framework that ensures consistency of results, making it easier for scientists to compare and share findings.
- Informing Policy Decisions: Assays are not just confined to laboratories; their outcomes influence regulatory policies and health standards. For example, environmental assays that measure pollutants directly impact legislation on air and water quality.
As stated in a recent study,
"The robustness of scientific assays directly correlates with advancements in technology and precision medicine, underscoring their importance in today’s research landscape."
Ultimately, understanding scientific assays is paramount for students, researchers, educators, and professionals alike. The future of science will rest on the reliable techniques that can only be refined through careful assay methodologies.
By delving into the intricate details of scientific assays, one not only appreciates the craftsmanship behind each procedure but also recognizes the pivotal role these methods play in advancing knowledge across various disciplines.
Historical Context
Understanding the historical context of scientific assays is crucial for grasping their evolution and significance in research and industry. As technologies advanced and scientific knowledge deepened, so did the methodologies employed to assess biological and chemical properties. Knowing where these methods come from brings to light not just their successes, but also the trials and errors that paved the way for innovation. The development of assays reflects a collective journey of human curiosity, precision, and the ethos of discovery.
Evolution of Assay Techniques
The journey of assay techniques has seen a remarkable transformation over centuries. Initially, basic observational techniques formed the bedrock of assessments in fields like medicine and agriculture. For instance, ancient herbalists relied on simple tests such as smelling or tasting herbs to determine their potency and effects on health. Moving into the 18th century, a gradual shift occurred. The advent of the scientific method introduced quantitative approaches that began to measure substances with precision.
In the late 19th and early 20th centuries, assays advanced significantly with the introduction of instrumentation. For example, the development of spectrophotometry allowed the quantification of light absorbance, revolutionizing how chemists could analyze solutions. This is when laboratory work shifted from mere observation to intricate calculations and reliable results. Modern assays now utilize techniques such as high-throughput screening which can analyze thousands of samples rapidly, capturing an unprecedented amount of data.
"The course of assay development illustrates a relentless pursuit of accuracy, enabling science to push boundaries and open new avenues for understanding."
Key Milestones in Assay Development
Milestones in the assay development timeline are landmarks that reflect not only technical advancement but also societal needs. One such milestone was the invention of the ELISA (enzyme-linked immunosorbent assay) in the 1970s. Designed for high sensitivity and specificity, it has become a cornerstone in fields from clinical diagnostics to biodefense, allowing detection of antigens and antibodies in complex mixtures with remarkable accuracy.
The emergence of polymerase chain reaction (PCR) technology in the 1980s constitutes another pivotal moment. PCR enabled researchers to amplify tiny amounts of DNA, transforming genetic studies and diagnostics overnight. This technology has broadly impacted not just assays but fields such as forensics and evolutionary biology.
In recent years, we’ve seen the integration of automation and artificial intelligence in assay design, improving both efficiency and accuracy. The rise of microfluidics, too, has offered a miniaturized platform for conducting chemical and biological assays, leading to faster results with less reagent waste.
As these milestones showcase, the evolution and critical advancements in assay techniques are a testament to humanity's drive to innovate, making them indispensable tools in the quest for knowledge.
Types of Scientific Assays
When we look at the vast landscape of scientific research, the term "assay" pops up like a beacon, guiding researchers through their investigative journeys. Understanding the different types of scientific assays is no small feat; it's crucial for anyone engaged in research or applications across varied fields. The various assay types help categorize how substances interact, how one can measure biological activity, or discern compositional qualities of a sample. Each assay brings its own toolbox of techniques tailored to answer specific questions.
Here's a quick rundown of what we're diving into:
- Biological Assays
- Chemical Assays
- Physical Assays
These distinctions are not just academic; they carry weight in results and conclusions that can affect real-world applications, from drug development to environmental assessments. Let's explore these three main types in detail.
Biological Assays
Biological assays can be likened to the motion of a pendulum—they swing back and forth between detecting activity and assessing potency. These tests are often used to determine how a substance affects living organisms or biological systems. Take, for instance, the widely-used cell viability assays. They help researchers identify the toxic effects of new compounds on cell cultures.
Some key elements to consider in biological assays include:
- Sensitivity and Specificity: These measures help in understanding how finely-tuned an assay is. A highly sensitive assay will detect even minute quantities of a substance, while specificity ensures it is not responding to unrelated components.
- Ethical Considerations: Frequently, biological assays can involve animal testing, which raises ethical concerns. Researchers are increasingly tasked with finding alternative methods to reduce animal use while still obtaining reliable data.
- Reproducibility: A significant aspect where many run into trouble. Reproducibility is needed to support findings, yet biological variability can sometimes lead to dissimilar results in repeated tests.
Overall, biological assays serve a pivotal role in determining how substances interact at the cellular level, influencing drug discovery and environmental impact studies.
Chemical Assays
As we transition into chemical assays, think of them as the Sherlock Holmes of the scientific world. These assays analyze chemical constituents and their interactions. Whether it’s determining the concentration of a component in a substance or understanding how two substances react under certain conditions, chemical assays are indispensable.
When contemplating chemical assays, consider:
- Quantitative vs. Qualitative: These assays can either quantify a substance’s concentration or identify its presence and basic characteristics without providing precise amounts. Knowing which is needed for your research is essential.
- Types of Chemical Reactions: From titrations to chromatographies, different techniques in chemical assays depend on the nature of the substances involved. A titration might be utilized to measure acidity, while chromatography helps in separating mixtures.
- Environmental Risk Assessment: Chemical assays play a crucial role in detecting pollutants in the environment. For instance, determining the level of heavy metals in soil or water is vital for public health and safety.
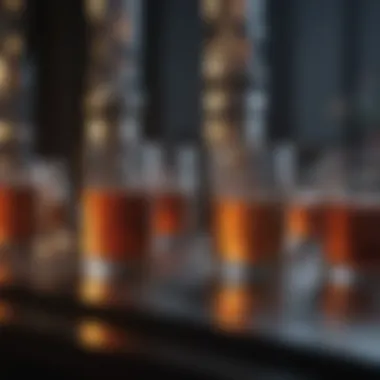
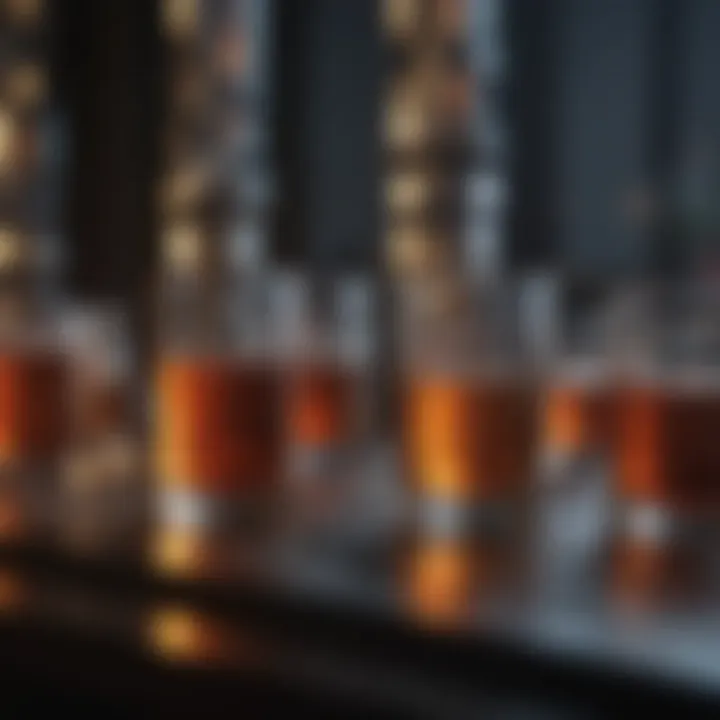
These facets underscore how chemical assays not only unravel the composition of substances but also provide a compass to steer our understanding of their interactions.
Physical Assays
Lastly, physical assays might not be famed like their biological and chemical counterparts, but they are the unsung heroes in the testing landscape. These assays often focus on measuring observable physical properties rather than biological or chemical interactions.
In physical assays, attention to detail matters. Here are a few examples:
- Spectroscopic Techniques: These methods analyze how light interacts with substances. Techniques like UV-Visible or Infrared spectroscopy help determine molecular structures and functionalities.
- Thermal Analysis: Methods like Differential Scanning Calorimetry (DSC) are used to understand how materials respond to changes in temperature, helping people figure out thermal stability and phase transitions.
- Mechanical Properties: In materials science, physical assays can assess elasticity, hardness, and tensile strength, which are critical for various applications, from construction materials to biomedical devices.
"Physical assays complement the understanding provided by chemical and biological tests, offering a holistic view of a sample's integrity and applicability in various scenarios."
In a nutshell, knowing the types of assays allows researchers and professionals to select the right approach for their specific needs, leading to accurate and relevant results across various fields.
Methodological Approaches
One cannot overstate the significance of methodological approaches in scientific assays. These approaches underpin the way researchers gather and analyze data, influencing the accuracy and reliability of their findings. Methodological rigor in assays ensures that outcomes are trustworthy and can be replicated, which is vital for the progress of science. In this section, we will dissect three key types of assays: quantitative, qualitative, and comparative. Each plays a unique role in the landscape of scientific inquiry.
Quantitative Assays
Quantitative assays serve as the backbone of many scientific investigations. They measure the amount of a specific substance in a sample, providing numerical data that can be analyzed statistically. The benefit of using quantitative methods is clear: they give objective figures that substantiate research claims. For instance, a pharmaceutical company might use a quantitative assay to determine the concentration of a drug in various formulations, ensuring consistency and efficacy.
One principal technique in quantitative assays is the ELISA (enzyme-linked immunosorbent assay), a method frequently used in labs to detect and quantify proteins in a sample. This method incorporates marked enzymes which produce a measurable signal, allowing for precise calculations of concentration.
Some key considerations when employing quantitative assays include:
- Calibration: Proper calibration of instruments is crucial to ensure accurate results.
- Replicates: Conducting multiple replicates can help confirm findings and minimize errors.
"In the realm of quantitative assays, consistency is critical. Trust the numbers and they will not fail you."
Qualitative Assays
On the flip side, qualitative assays offer insights into the presence or characteristics of substances without providing numerical data. They are indispensable in fields where understanding the nature of a sample outweighs the need for precise measurements. For example, a researcher may perform a qualitative assay to identify the types of bacteria present in a contaminated water sample, focusing on whether specific pathogens are present rather than quantifying their abundance.
During the process of qualitative assessment, methods such as staining, culturing, or visual inspection are commonly employed. These approaches allow for the evaluation of traits such as color change, growth patterns, or even fluorescence. While qualitative methods do not provide the granular data that quantitative assays do, they can quickly signal if further quantitative analysis is warranted.
Key aspects to keep in mind concerning qualitative assays involve:
- Sensitivity: Qualitative assays often have high sensitivity to detect low concentrations of compounds, making them valuable in early-stage research.
- Subjectivity: The interpretation of results may vary, necessitating a clear and consistent methodology to draw valid conclusions.
Comparative Assays
The third type, comparative assays, are essential in understanding differences and similarities between various samples or treatments. These especially shine in experimental setups where researchers want to assess the impact of different variables on their subjects. For instance, researchers may compare the effectiveness of two different drugs on the same type of cancer cells.
A comparative assay might employ both qualitative and quantitative deductions to draw comprehensive conclusions. By juxtaposing results from various conditions, scientists can elucidate trends and make informed recommendations about which treatments are most effective.
Some critical considerations in comparative assays include:
- Controls: Adequate control groups are vital to make meaningful comparisons. Without these, it’s easy to misinterpret results.
- Statistical Validity: Proper statistical analysis is necessary to ensure that observed differences are significant and not due to random chance.
Assay Development and Design
Assay development and design serve as the backbone of successful experimental research. Without a robust design, the entire process can unravel, leading to incorrect conclusions, wasted resources, and time lost. While the scientific community values the end results of assays, the journey to those results is equally important. This section dives into the elements that comprise effective assay design, focusing on scoping objectives, selecting appropriate reagents and controls, as well as ensuring optimization and validation. Each aspect plays a crucial role in producing reliable and reproducible data in various disciplines.
Scoping and Objectives
Scoping and objectives lay the groundwork for all assay development. Think of this stage as the blueprint for a house; without it, you risk building something that doesn’t meet your needs. Here, researchers need to ask critical questions: What is the goal of the assay? What specific parameters should be measured? Establishing clear objectives not only guides the design process but also ensures that the end results answer the research question effectively.
- Defining the Purpose: Clarity in purpose helps prevent scope creep. This means recognizing the difference between exploratory and conclusive assays.
- Target Audience: Considering who will use the results can also shape how an assay is designed. For instance, will it be for academic purposes, regulatory submission, or internal quality control?
In short, take time to lay out the scope and objectives before diving into the nitty-gritty. It is a crucial step to avoid pitfalls later in the assay development process.
Reagents and Controls
Selecting reagents and controls is a vital part of assay design that directly influences the reliability of results. The choice of reagents must align with the assay objectives, and currently known variables must be accounted for to ensure accuracy in findings.
Key considerations include:
- Quality of Reagents: Always opt for high-quality, well-characterized reagents. Poor quality substances may lead to equivocal results, mudding the waters of data interpretation.
- Control Samples: Controls help validate results. This includes positive controls, which should show expected results, and negative controls, which ensure that results are due solely to the intended reactions, eliminating false positives.
A comprehensive selection of reagents combined with the appropriate controls can significantly enhance the reliability of the outcomes, effectively guiding further research or applications.
Optimization and Validation
In the world of assays, optimization and validation go hand in hand. Each assay has its quirks; some may require tweaks in temperature, pH levels, or even incubation times to yield the best outcomes. This iterative process of fine-tuning is essential for creating an efficient assay.
- Optimization: During this phase, factors affecting assay performance are systematically varied to find the best operational conditions. The goal is to enhance sensitivity, specificity, or speed, depending on the assay's intended outcomes.
- Validation: Once the assay is optimized, validation follows. This process confirms that the assay consistently delivers accurate and reproducible results. It often involves running a series of tests and comparing the outcomes against established standards.
Incorporating optimization and validation procedures into assay design is not merely a formality; it is essential for producing credible and actionable data.
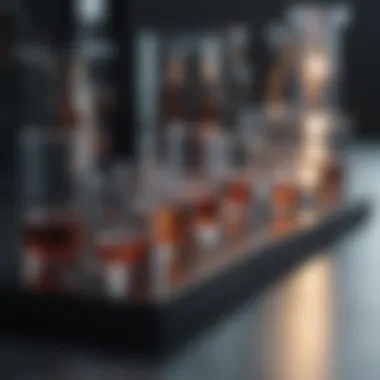
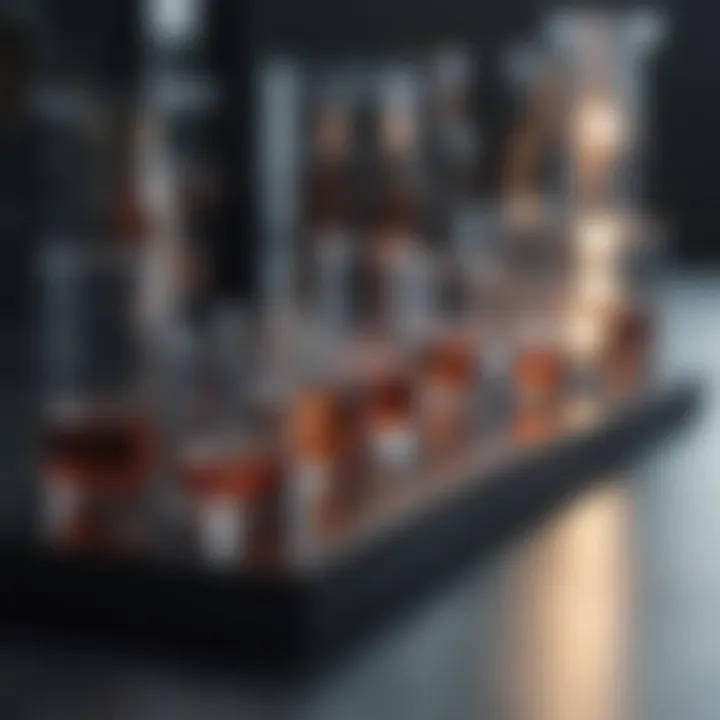
"An assay that is poorly designed is like a ship without a rudder; you may have all the materials on board, but you’ll find yourself adrift when it matters most."
By focusing on these stages of assay development, researchers can craft a detailed roadmap leading to reliable outcomes. Whether the end goal is regulatory compliance, academic publication, or technological advancement, a well-designed assay withstands scrutiny and ultimately contributes meaningful data to the scientific community.
Applications of Scientific Assays
Scientific assays are pivotal in advancing various fields, as they provide the framework for testing hypotheses and evaluating outcomes. These methodologies enable researchers to unravel complex biological mechanisms, assess environmental conditions, and diagnose clinical conditions. Understanding the applications of scientific assays can illuminate their significance and utility in shaping research and industry practices.
Pharmaceutical Industry
The pharmaceutical industry stands as one of the most prominent sectors that leverage scientific assays. In drug development, assays play an integral role in the discovery and optimization of pharmaceutical compounds. Early phases involve high-throughput screening using automated systems that can test thousands of compounds for biological activity. This allows researchers to efficiently identify leads that possess desired therapeutic properties.
Consider the importance of validating drug efficacy. Assays help in determining whether a drug interacts with its target as intended. The process doesn’t end with efficacy; safety assessments are crucial too. Toxicology assays help in understanding the potential adverse effects of a drug, ensuring that only the safest candidates advance to clinical trials.
Moreover, assays are employed in pharmacokinetics and pharmacodynamics to evaluate how drugs behave in the body. For example, they can measure how a drug is absorbed, distributed, metabolized, and excreted. These assessments directly impact dosage regimens and therapeutic guidelines, ultimately fostering more effective patient care.
Environmental Monitoring
The significance of scientific assays in environmental monitoring cannot be overstated. As societies face increasing environmental challenges, assays become essential tools for maintaining ecological integrity. Through various chemical assays, toxic substances in soil and water can be detected, quantifying pollutants and assessing their impact on ecosystems.
For instance, biosensors facilitate the detection of contaminants, allowing for rapid analysis of environmental samples. These assays go a long way in tracking pollutants from industrial runoff or agricultural practices, offering a way to hold industries accountable for their environmental footprint. Correspondingly, biological assays help in assessing the health of ecosystems by examining indicator species. By monitoring changes in the populations of these organisms, researchers can infer the overall health of an environment.
Environmental regulations are often formed based on data obtained from such assays, making them indispensable for policymakers and researchers alike.
Clinical Diagnostics
In clinical diagnostics, scientific assays are the bedrock for disease detection and monitoring. They provide critical data that aids in the diagnosis of various health conditions—from infectious diseases to genetic disorders. Molecular assays, such as PCR (polymerase chain reaction), have transformed how diseases like COVID-19 are diagnosed, providing fast and reliable results that prove essential in managing public health.
The role of serological assays is also crucial here, where they test for antibodies or antigens to show whether an individual has been exposed to particular pathogens. These assays can help in epidemiological studies, giving insights into the spread of diseases in populations.
Moreover, continual advancements in precision medicine underscore the relevance of assays in tailoring treatments based on an individual’s specific condition. By employing assays that determine the genetic makeup of a person's cancer, healthcare professionals can provide targeted therapies that are more effective and less toxic than traditional treatments.
"Scientific assays not only pave the way for medical discoveries but also embed themselves into the ethos of responsible health management."
In summary, the applications of scientific assays range across various industries, bolstering advancements in pharmaceuticals, enhancing environmental management, and refining clinical diagnostics. Their significance is rooted in their ability to generate reliable data that supports evidence-based decisions and fosters innovation.
Technological Advances in Assays
Scientific assays have come a long way since their inception, evolving into sophisticated methodologies that leverage advanced technologies. These advances are pivotal, as they not only enhance the precision of results but also improve efficiency and scalability in various fields from pharmaceuticals to environmental monitoring. It’s through these technologies that researchers can tackle more complex problems and extract valuable insights faster than ever before.
High-Throughput Screening
High-throughput screening (HTS) is often seen as a game changer in the drug discovery landscape. It allows for the rapid testing of thousands of compounds against targeted biological processes. By automating the process, researchers can evaluate the effects of numerous substances simultaneously, which means more data in shorter times.
The significance of HTS lies in its ability to streamline the drug development pipeline. Traditional methods of testing drugs can take years and cost millions; however, HTS can fast track this, identifying potential leads within days. Every hit identified is a stepping stone towards effective therapies for diseases that currently have limited treatment options.
Microfluidics
Microfluidics plays an essential role in the modern laboratory, allowing manipulation of fluids at microscopic scales. This technology can produce minute amounts of samples and reagents, making it economical and reducing waste which is vital considering environmental impacts. The precision of microfluidic devices can lead to more accurate assay results, enabling researchers to make predictions about biological behavior with greater confidence.
Moreover, microfluidics allows for the integration of multiple assays on a single chip, leading to multiplexing capabilities. Imagine needing only one drop of blood to run various tests simultaneously; this not only saves precious time but also resources. Researchers are now able to design experiments more creatively, hence opening doors for new discoveries.
Automated Assay Systems
Automated assay systems represent another advancement that propels the scientific community forward. These systems eliminate human error and enable continuous operation, which is indispensable in high-demand environments like pharmaceutical companies. With systems that can carry out repetitive tasks, analysts are freed up to do what they do best—think critically and innovate.
Automation can also enhance the reproducibility of assays. Precision equipment can generate consistent results over time, making findings more reliable. This is especially crucial when data will be used to inform clinical decisions or regulatory submissions. It's also worth noting that, as these systems become more sophisticated, they often incorporate advanced data analytics capabilities, paving the way for real-time analysis and insights.
"The advent of automation in assay systems is not merely a trend; it’s an essential evolution for achieving accurate and reproducible results in an increasingly complex scientific landscape."
In summary, the technological advances in assays are crucial for the future of scientific research. With methodologies such as high-throughput screening, microfluidics, and automated systems, researchers are better equipped to meet the demands of modern science. These advancements not only increase efficiency and reliability but also stimulate creativity in research applications. The possibilities are vast, and as technology evolves, so too will our ability to innovate and discover.
Regulatory and Ethical Considerations
Scientific assays, while crucial in advancing knowledge across various disciplines, bring forth a host of regulatory and ethical implications that are essential for researchers to grasp. Adhering to established rules not only ensures the credibility of results but also protects the rights of individuals and the environment involved in assay processes. This segment illuminates the significance of these considerations and underscores the necessity of maintaining high standards in scientific inquiry.
Compliance Standards
The landscape of scientific research is governed by a myriad of compliance standards designed to uphold the integrity of assays. These standards serve multiple purposes:
- Protecting Human and Animal Welfare: Regulations, like the Animal Welfare Act, ensure that research involving living organisms is conducted ethically and humanely. Adapting methods that reduce suffering is not just a legal obligation; it's a moral one.
- Quality Assurance: Compliance standards like Good Laboratory Practice (GLP) and Good Manufacturing Practice (GMP) dictate consistent processes. This minimizes errors and ensures that assay results are reproducible, which is vital for further research and application.
- Environmental Impact: Environmental assessments often dictate methodologies in assays, especially in chemical analysis. Researchers must consider potential contamination and take measures to mitigate environmental damage, complying with regulations such as the Environmental Protection Agency (EPA) guidelines.
Maintaining compliance often involves extensive documentation that reflects the methodologies applied, the calibration of equipment, and the handling of reagents. > "In science, paper trails are as critical as the experiments themselves. They offer accountability and transparency."
Ethical Issues in Assays
Despite the frameworks setting the boundaries, ethical issues can still obscure the pathway of integrity in assay-related work. Here are some pivotal concerns:
- Informed Consent: In studies involving human subjects, it is imperative to obtain informed consent. Individuals must understand how their data will be used and the potential risks involved.
- Data Fabrication and Falsification: The pressure to produce positive results can lead to unethical practices like manipulating data. This practice not only misleads peer reviewers but also endangers future research that builds upon false premises.
- Intellectual Property Rights: The ownership of assay technology and methods often leads to disputes. Researchers must navigate these waters carefully to respect the contributions of all involved.
- Cultural Sensitivity: In some instances, assays may intersect with cultural beliefs, especially in clinical studies. Researchers ought to approach these topics with respect, ensuring that they do not offend or exploit communities.
The intersection of science with ethics is no small matter. Understanding and adhering to these ethical issues forms the backbone of responsible research and fosters trust within the communities impacted by scientific advancements.
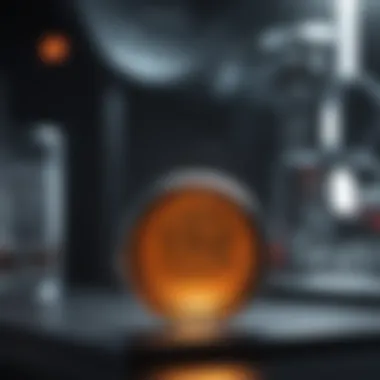
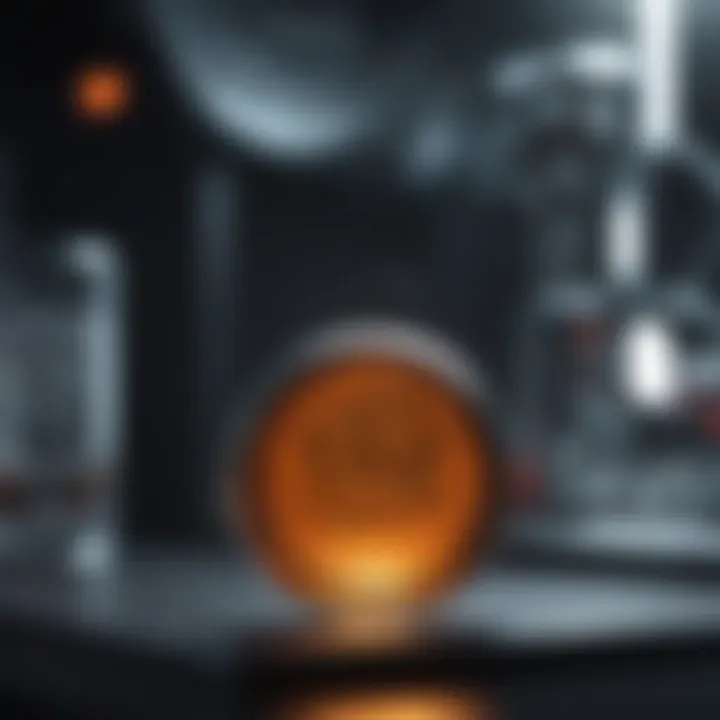
Data Analysis in Assays
Data analysis plays a quintessential role in the realm of scientific assays. The way data is handled post-experiment can determine the validity of the results, influence subsequent scientific claims, and shape future research directives. It’s not merely about crunching numbers; it’s about distilling meaningful insights from complex datasets. The aim here is to transform raw, unrefined data into clear, actionable conclusions.
One specific benefit of effective data analysis is that it brings order to chaos. In the lab, researchers may generate terabytes of information from a single series of assays, especially in high-throughput settings. Proper analysis techniques allow for the distillation of this information into comprehensible patterns and trends. This clarity is paramount when communicating findings to the broader scientific community or stakeholders, as it underpins the overall reliability of the study.
Statistical Techniques
In the toolkit of assay data analysis, statistical techniques serve as the backbone. Researchers employ various statistical methods to assess the significance of their results, compare them against existing data, and ultimately draw relevant conclusions. Some of the key statistical techniques used in this context include:
- Descriptive Statistics: These provide basic insight into the data—mean, median, mode, and standard deviation help in understanding the general trends of the dataset.
- Inferential Statistics: These techniques allow scientists to make predictions or assumptions about a larger population based on a small sample. T-tests, ANOVA, and regression analysis are some common methods here.
- Multivariate Analysis: When dealing with multiple variables, multivariate techniques can shine a light on interactions or relationships that are not immediately apparent. Principal component analysis and cluster analysis can be especially illuminating.
It’s important to remember that the choice of statistical methods can significantly affect the interpretation of assay data. A common pitfall might be using the wrong test without validating the underlying assumptions. This could lead to faulty conclusions, which in the world of science, can be more than just a minor error—it can have cascading repercussions.
Interpreting Assay Results
Once data has been gathered and subjected to rigorous statistical analysis, the next step is interpretation. This phase demands both skill and experience, as it involves translating numerical results into meaningful scientific narratives. Here, the challenge is to avoid the trap of over-interpreting or cherry-picking data to fit preconceived notions or hypotheses. The following considerations often guide the effective interpretation of assay results:
"The meaningfulness of data is not merely in its numbers but in how those numbers weave into the broader tapestry of scientific understanding."
- Contextual Relevance: Results must be considered within the context of the study. What was the initial hypothesis? How do these results align with or diverge from previous findings?
- Statistical Significance vs Practical Significance: A result can be statistically significant yet practically insignificant. It’s crucial not only to acknowledge statistical outcomes but also to assess their real-world applicability.
- Reproducibility: Solid interpretation should lead to findings that are reproducible under the same conditions. Results that cannot be replicated call for skepticism.
In summarizing assay results, it is essential to clearly communicate limitations and uncertainties. The scientific method thrives on transparency, and admitting where a study falters can pave the way for future exploration and understanding. Ultimately, effective data analysis and interpretation will ensure that the findings contribute meaningfully to the scientific community and its ongoing inquiry into pressing challenges.
Challenges and Limitations
When discussing scientific assays, it’s vital to delve into the challenges and limitations that researchers often encounter. These aspects significantly shape assay design, execution, and the reliability of the results gathered. Understanding these hurdles not only aids in the improvement of existing assays but also steers new developments in assay science. By recognizing potential pitfalls, researchers can devise effective strategies and methodologies to navigate these challenges, consequently enhancing the quality and applicability of their work.
Common Pitfalls in Assay Design
The design of an assay is much like crafting a recipe - if you miss a step or use the wrong ingredient, the dish might not turn out as expected. Common pitfalls in assay design can lead to flawed results, which can derail an entire research project. Here are some of the crucial mistakes to be aware of:
- Inadequate Planning: Many researchers jump straight into assays without a thorough understanding of objectives and parameters. This can lead to an unstructured approach, where important variables are overlooked.
- Poor Choice of Controls: Utilizing inappropriate or insufficient control samples can cloud the interpretation of results, making it tough to discern whether observed effects stem directly from the test conditions.
- Overlooking Sample Preparation: Neglecting the importance of sample integrity during preparation can introduce contaminants or degrade the samples, ultimately skewing results.
- Ignoring Replicates: Failing to include sufficient replicates can result in data that’s not robust, leading to generalizations that might not hold true across broader contexts.
Addressing these common pitfalls requires a meticulous approach to assay design. Researchers should establish clear objectives and select controls wisely, considering the overarching context of their studies. This awareness can pave the way for more accurate and reproducible results.
Limitations of Existing Assays
Every tool has its limitations, and scientific assays are no different. While existing assays have paved the way for immense advancements in various fields, recognizing their limitations is crucial for future innovation. Some inherent restrictions include:
- Sensitivity Issues: Many assays may not be sensitive enough to detect low-abundance targets, which is particularly critical in areas like clinical diagnostics where early detection can significantly alter outcomes.
- Specificity Limitations: Some assays struggle with specificity, leading to false positives or negatives. This is a significant hurdle when applying results to real-world scenarios, particularly in biomedicine.
- Scale Limitations: Assays developed on a smaller scale might not translate well to larger or different populations, limiting their applicability.
- Cost-Effectiveness: Advanced assays can often be costly and time-consuming to perform. This can deter widespread implementation in smaller labs or resource-limited settings.
Recognizing these limitations serves as a critical impetus for researchers and developers to innovate and refine current methodologies. It can lead to the development of next-generation assays that are not only more sensitive and specific but also more practical for varied applications.
"Understanding the limitations of existing technologies is the first step toward true innovation."
Future Directions in Assay Research
The field of scientific assays is on the precipice of significant advancements. As we explore future directions in assay research, it's critical to understand that these developments are not just a matter of improvement, but transformation. Enhancements in accuracy, speed, and efficiency are continuously shaping the landscape of various disciplines, including biotechnology, pharmaceutical development, and environmental monitoring.
Innovative Assay Concepts
In recent years, researchers have begun to think outside the box, conceptualizing assays that leverage cutting-edge technologies. For instance, the integration of nanotechnology in assay development has opened up new avenues for detection and quantification at molecular levels. From biosensors that can detect pathogens with extreme sensitivity to point-of-care testing devices that deliver rapid results, these innovations promise to revolutionize diagnostics and monitoring.
Moreover, the rise of lab-on-a-chip technology is transforming how assays are performed. These miniaturized devices can conduct multiple biochemical reactions simultaneously, such as measuring drug responses in real-time. This not only narrows down experimental timelines but also minimizes the use of reagents, which brings cost savings.
"The future is here, and it’s not evenly distributed yet." - William Gibson
As we further our understanding in this space, it's essential to consider the implications of adopting AI and machine learning in assay design. By crunching vast amounts of data, predictive algorithms can streamline the development process, enabling researchers to pinpoint potent compounds more efficiently. Consequently, innovative assay concepts are not just about new techniques; they also focus on creating a synergy between technology and biology, which is vital for progress.
Interdisciplinary Approaches
The nature of scientific inquiry is inherently interdisciplinary. Moving forward, assay research can benefit greatly from collaboration among various scientific fields. For instance, combining molecular biology with data science promises to create more robust methods for assay analysis. By intertwining these disciplines, researchers can cultivate a comprehensive framework that captures both biological nuances and computational efficiencies.
Furthermore, addressing complex global challenges such as climate change or disease outbreaks requires a suite of expertise. Assay development can integrate insights from environmental science, epidemiology, and public health, leading to more holistic approaches. An example can be seen in environmental forensic assays—assays that draw on techniques from chemistry, biology, and statistics to identify pollutants in ecosystems.
Additionally, collaborative research efforts across universities, research institutions, and industries accelerate the pace of innovation. Establishing partnerships can help leverage shared resources, expertise, and technology. This direction fosters an ecosystem that embraces diverse skills and perspectives, which is essential for tackling intricate issues in assay research.
As we look towards the future, emphasizing innovative assay concepts alongside interdisciplinary collaboration paves the way for a robust experimental framework that not only meets current demands but is also flexible enough to address emerging challenges in science and society.
End and Summary
In synthesizing the information elaborated through this article, it becomes clear that scientific assays serve as crucial instruments within various research landscapes. Their capacity to provide repeatable and reliable measurements significantly contributes to the integrity of scientific inquiries. Assays function as the backbone for advancements in pharmaceutical development, environmental monitoring, and clinical diagnostics; they not only facilitate the understanding of complex biological systems but also enhance the decision-making process in research and industry settings.
Key Takeaways
- Diversity of Assays: Scientific assays encompass a wide range of techniques, from biological to chemical and physical methods, each tailored for specific research needs and objectives.
- Methodological Rigor: The design of an assay requires clear objectives, properly chosen reagents, and extensive validation processes to ensure accuracy and reproducibility of results.
- Technological Integration: Modern advances such as high-throughput screening and automated systems have revolutionized the landscape of assays, making them more efficient and accessible than ever before.
- Ethical Considerations: Despite their significance, the development and application of assays must adhere to regulatory standards and ethical guidelines to mitigate potential negative impacts on both human subjects and the environment.
"Scientific assays are the linchpins of modern research, bridging the gap between theoretical inquiry and practical application."
- Challenges and Limitations: Researchers must remain aware of the inherent limitations of existing assays, which may affect result interpretation and applicability within varied contexts.
Implications for Future Research
The trajectory of research surrounding scientific assays is poised for significant evolution. As scientists continually seek innovative methods to enhance assay sensitivity and specificity, interdisciplinary approaches will gain traction. This may involve collaborations between fields such as bioengineering, nanotechnology, and computer science. For instance, integrating artificial intelligence could streamline data analysis, leading to more nuanced interpretations of assay results.
Moreover, environmental and societal demands will likely drive the development of assays focused on sustainability and ethical use of resources. An increased emphasis on green chemistry, for example, could shape the approach to reagent selection and assay development in the years ahead. Additionally, the responsiveness of assay technologies to emerging health crises reiterates the importance of adaptability in research design, aiming to address pressing global challenges effectively.
With continuous advancements in technology, shifting regulatory frameworks, and changing societal needs, the future of scientific assays promises to be dynamic and multifaceted, opening doors to novel discoveries and methodologies.