How Genes Are Sequenced: An In-Depth Exploration
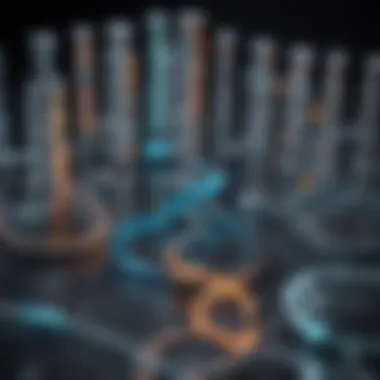
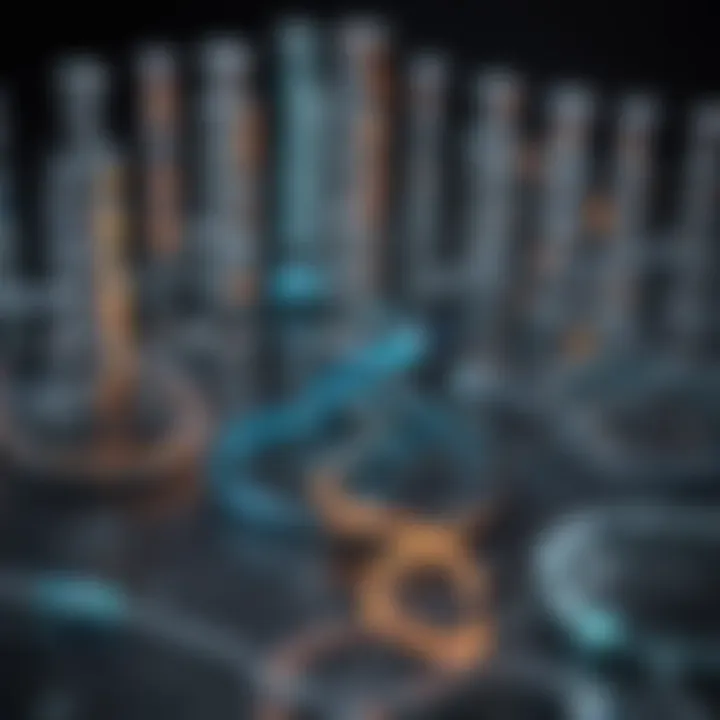
Intro
Gene sequencing is a fundamental process in genetic research. It involves determining the precise order of nucleotides in a DNA molecule. With the advent of various technologies, gene sequencing has evolved from basic methodologies to sophisticated techniques that enable researchers to decode genomes efficiently. This article explores the essential aspects of gene sequencing, offering insights into its history, methodologies, and the significance of its advancements.
Research Overview
Summary of Key Findings
The exploration of gene sequencing reveals several critical findings:
- Historical Evolution: The journey of gene sequencing began in the 1970s with the advent of Sanger sequencing. Over the decades, it has greatly changed with the introduction of Next-Generation Sequencing (NGS) techniques.
- Methodological Diversity: Different methods exist, including Sanger sequencing, Illumina sequencing, and Nanopore sequencing. Each of these methods has its unique advantages and challenges.
- Technological Impact: Advancements in sequencing technology have drastically reduced costs and improved accuracy in genetic studies.
Significance of Research
Understanding gene sequencing is vital for various fields, including medicine, agriculture, and evolutionary biology. Discoveries in genetic sequencing contribute to personalized medicine, disease research, and the development of genetically modified organisms. The implications extend into ethical discussions as well, providing necessary context for a rapidly evolving field.
Methodology
Research Design
The research encompassed a review of historical documents, analysis of current sequencing methodologies, and the examination of case studies demonstrating the application of sequencing technologies.
Data Collection Methods
Data collection involved qualitative methods, including literature reviews and expert interviews. These approaches aimed to gather comprehensive insights about the development and implications of gene sequencing.
Gene sequencing is a continually evolving field, and this article aims to shed light on its intricate nature and broad implications on science and society. Understanding its fundamentals is essential for students, researchers, educators, and professionals looking to navigate the complexities of genetic research.
Prelude to Gene Sequencing
Gene sequencing is a fundamental aspect of modern biology and medicine. It allows scientists and researchers to decode the genetic blueprint of living organisms. By understanding genes, their structure, and their functions, we unlock insights into evolutionary processes, diseases, and the complexities of life itself. This section will discuss the significance of gene sequencing in advancing our knowledge in various fields, including genetics, medicine, and biotechnology.
Gene sequencing involves determining the precise order of nucleotides in a DNA molecule. Each gene carries instructions that govern physical traits and biological functions. The ability to sequence these genes has far-reaching implications, such as identifying genetic disorders, developing targeted therapies, and enhancing agricultural practices. Essentially, gene sequencing serves as a tool for exploration and innovation, driving forward scientific inquiry and application.
Furthermore, gene sequencing has benefits beyond research. In medicine, it provides the basis for precision medicine, where treatments are tailored to individual genetic profiles. Additionally, understanding genetic variability can inform public health strategies and disease management.
Thus, gene sequencing stands as a cornerstone of genetic research, offering a glimpse into both the mechanics of life and the potential for groundbreaking advancements in health and science.
Understanding Genes and Their Importance
Genes are segments of DNA that play an essential role in determining the characteristics of living organisms. Each gene functions as a code, instructing cells on how to produce proteins, which are vital for development, structure, and function. The importance of genes lies in their influence on heredity, genetic diversity, and evolution.
In the human context, genes can affect personal traits such as eye color and susceptibility to diseases. For scientists, understanding these genes is crucial for researching genetic conditions and creating effective treatments. By investigating genetic mutations and their impacts, researchers can explore complex biological questions and develop solutions for health issues.
It is also important to consider the ethical dimensions of gene research. The ability to manipulate genes raises questions about privacy, consent, and the potential for misuse of genetic information. As gene sequencing technology progresses, so too must our understanding of the responsibilities that come with it.
Brief History of Gene Sequencing
The journey of gene sequencing began in the mid-20th century with the discovery of the DNA structure by James Watson and Francis Crick. Their work paved the way for understanding how genetic information is stored and transmitted.
The first significant leap in gene sequencing came with the introduction of Sanger sequencing in the 1970s. Developed by Frederick Sanger, this method allowed scientists to determine the order of nucleotides in a DNA segment accurately. Over the next few decades, advancements in automated sequencing technologies accelerated the process and reduced costs.
In the early 2000s, the Human Genome Project represented a monumental achievement. This international collaboration aimed to sequence the entire human genome, and it was completed in 2003. The data generated from this project has been seminal in advancing genetics research.
Today, new techniques like Next-Generation Sequencing (NGS) continue to revolutionize the field, enabling the sequencing of even complex genomes at unprecedented speeds. Each advancement has expanded our knowledge base and enhanced our ability to address critical scientific and medical questions.
Sequencing Technologies Overview
Understanding the various sequencing technologies is essential in the context of gene sequencing. Each technology has distinct advantages, making them suitable for specific applications in research and clinical settings. This overview aims to elucidate the fundamental differences among these methods, their historical significance, and the innovative approaches they bring to genetic research.

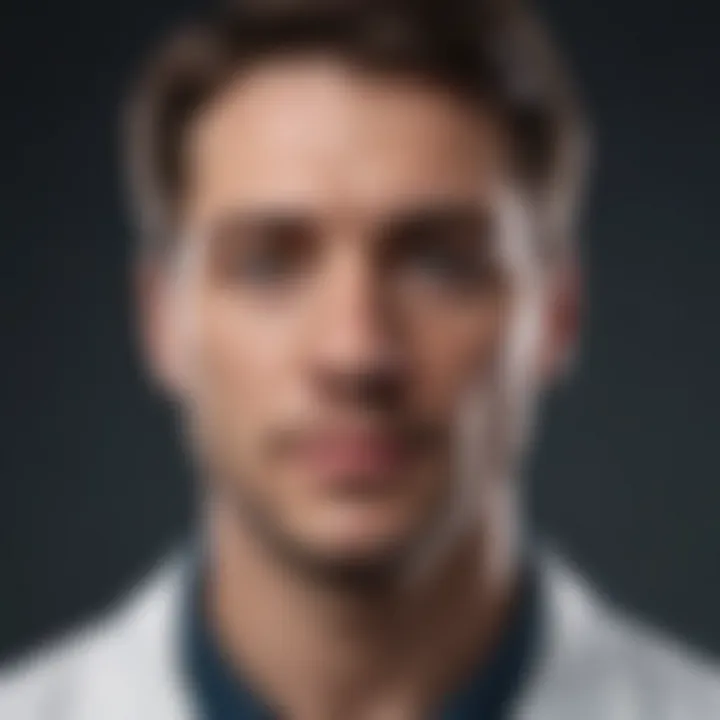
Sanger Sequencing
Sanger Sequencing, developed by Frederick Sanger in the 1970s, is often regarded as the first reliable method of DNA sequencing. It employs dideoxynucleotides, which terminate DNA strand elongation during replication, allowing researchers to determine the nucleotide sequence effectively. The main advantages of Sanger Sequencing include its high accuracy and reliability, which made it the gold standard for many years.
One critical aspect of Sanger Sequencing is its application in verifying sequences obtained from Next-Generation Sequencing methods. Its simplicity lends itself well to smaller-scale projects and sequencing of specific genes. Nevertheless, there are some limitations. Sanger Sequencing is relatively slow and can be costly for larger-scale sequencing since it typically processes only one DNA fragment at a time.
Next-Generation Sequencing (NGS)
Next-Generation Sequencing represents a groundbreaking shift in sequencing technology, enabling rapid and cost-effective analysis of genomic information. Unlike Sanger Sequencing, NGS can simultaneously sequence millions of fragments, allowing for what is often referred to as multiplexing. One of its primary benefits is its ability to generate vast amounts of data in a significantly shorter period.
NGS applications are diverse, ranging from large genomic studies to targeted panels for specific diseases. Many researchers utilize this technology for whole-genome sequencing, targeted resequencing, and transcriptome analysis. However, NGS also has its challenges. Data management is a critical concern, as the volume of information produced is substantial. Moreover, while NGS is known for its throughput, the accuracy can be lower than Sanger due to sequencing errors that are more likely in highly repetitive regions of the genome.
"Next-Generation Sequencing has revolutionized the way we approach genetic research by allowing unprecedented access to genomic data."
Third-Generation Sequencing
Third-Generation Sequencing brings forth an advanced approach to genomic analysis. It introduces single-molecule sequencing technologies, which allow for the sequencing of DNA without the need for amplification. Techniques like PacBio's SMRT (Single Molecule Real-Time) sequencing and Oxford Nanopore's technology exemplify this category.
These methods provide significant benefits, such as longer read lengths and the ability to perform real-time sequencing. The advantage of longer reads enables better assembly of complex genomes and improved detection of structural variants. Furthermore, because these technologies do not require amplification, the introduction of biases during this step is eliminated, enhancing the accuracy of sequencing data.
However, Third-Generation Sequencing is still evolving. The costs have been decreasing, but they remain higher compared to NGS. Moreover, issues with data quality need further refinement as this technology becomes more widely implemented in research and clinical applications.
In summary, each sequencing technology plays a pivotal role in advancing our understanding of genetics. Sanger Sequencing continues to provide reliable results for specific applications, while Next-Generation Sequencing and Third-Generation Sequencing push the boundaries of what is possible in genomic research. These technologies collectively contribute to our comprehension of genetic information and its practical implications.
The Gene Sequencing Process
The gene sequencing process is a critical aspect of understanding genetic information. It is essential for various applications, such as clinical diagnostics and personalized medicine. Good sequencing processes ensure high-quality data, which is necessary for accurate results. This leads to significant advancements in medical research, agriculture, and genetic studies.
Sample Preparation
Sample preparation is the foundation of the sequencing process. It involves several steps designed to isolate the DNA from cells and ensure that the sample is suitable for sequencing.
DNA Extraction
DNA extraction is the initial step in sample preparation. This process involves breaking down the cellular structure to release the DNA. A key characteristic of DNA extraction is its versatility; it can be performed using various methods, including chemical lysis and mechanical disruption. This flexibility makes extraction favorable for different types of samples, ranging from tissues to blood.
The unique feature of DNA extraction is that it reveals the integrity of the DNA. High-quality DNA extraction is vital because degraded DNA can lead to inaccurate sequencing results. However, one disadvantage is that the extraction process can be time-consuming and may require specific protocols depending on the sample type.
Quality Control
Quality control follows DNA extraction. It ensures that the extracted DNA is of sufficient quality and quantity for sequencing. Quality control is crucial because it validates the sample before it moves to the next stage. Techniques like gel electrophoresis and spectrophotometry are common in this phase.
The unique feature of quality control is its ability to catch potential errors early in the sequencing process. This proactive measure is beneficial, as it saves time and resources in the long run. However, quality control can sometimes introduce additional costs and requires specialized equipment.
Library Preparation
Library preparation is another critical step in the sequencing workflow. It involves formatting the DNA in a way that allows for effective sequencing.
Fragmentation
Fragmentation involves breaking the DNA into smaller pieces. This is important because many sequencing methods require DNA of certain lengths for optimal results. This step is popular due to the need for consistency in fragment sizes, which ensures better coverage in sequencing runs.
The unique feature of fragmentation is the compromise between fragment size and yield. While shorter fragments can improve coverage, they may yield less overall DNA. This complexity makes fragmentation a careful balancing act.
Adapter Ligation
Adapter ligation is the next phase in library preparation. It entails attaching short, known sequences of DNA, called adapters, to the ends of fragmented DNA. A key characteristic of adapter ligation is that it allows the sequencing machine to recognize and bind the DNA fragments effectively.
This step facilitates higher efficiency in sequencing through amplification. However, one should note that improper ligation can lead to low sequencing quality, affecting the overall data.
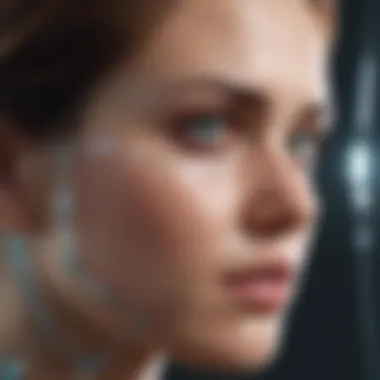
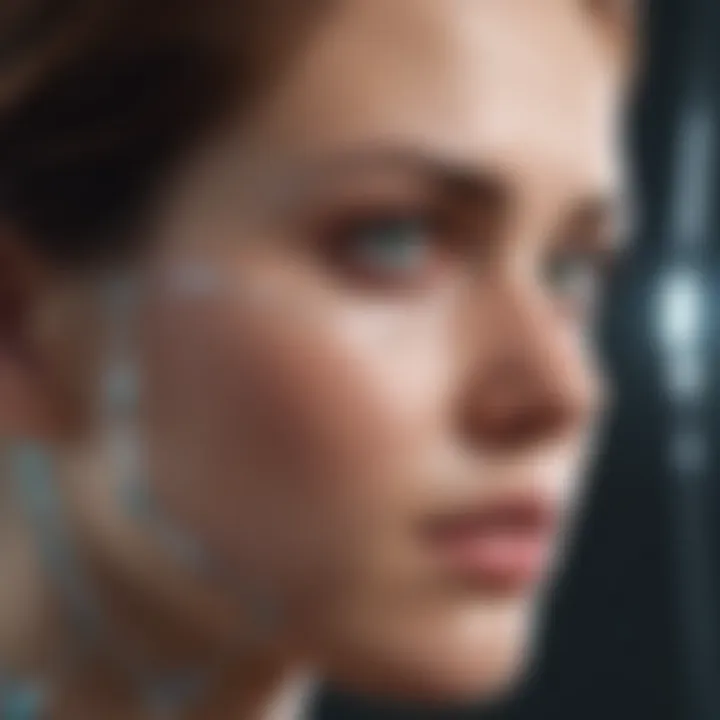
Sequencing Execution
Once the samples are prepared, sequencing execution occurs. This is where the actual sequencing takes place, and it is arguably the most technical aspect of the process.
Platform-Specific Protocols
Different sequencing platforms have specific protocols for execution. These protocols guide how to introduce the samples to the sequencer. This specificity is vital as each machine may operate on different principles.
The unique aspect of platform-specific protocols is careful adherence. Following the exact protocol ensures optimal performance, yet this can vary significantly in complexity from platform to platform, leading to potential errors if misunderstood.
Data Acquisition
Data acquisition is the collection of sequencing data generated by the machines. A key characteristic of this step is its dependence on high-throughput capabilities of modern sequencers, which can generate vast amounts of data quickly.
This should allow for broad applications across many fields. However, with large data sets comes the challenge of data storage and management, which can be a disadvantage in terms of resource allocation.
Data Analysis
Data analysis interprets the raw sequencing data into meaningful information. This is a multifaceted process that holds equal importance to all preceding steps.
Sequence Alignment
Sequence alignment is the first step in data analysis. It compares the sequenced fragments against a reference genome. The key characteristic of sequence alignment is its ability to identify where the sequences match or differ.
This step is critical for accurate variant detection and is a well-established method in bioinformatics. However, it can be computationally intensive, requiring substantial processing power.
Variant Calling
Variant calling follows sequence alignment and involves determining the genetic variants present in the sample. This step is beneficial as it informs researchers about mutations that may influence disease risk or treatment efficacy.
The unique feature of variant calling is the complex algorithms that underpin this analysis, which may vary in accuracy based on the sequencing method. A disadvantage is that noise in the data can lead to false positives, requiring careful interpretation.
Applications of Gene Sequencing
Gene sequencing has transcended its initial role of merely elucidating the structure of DNA. Today, it has vital applications across various fields. Its importance lies in how it can directly benefit health, agriculture, and even our understanding of biological systems. This section delves into the pivotal areas where gene sequencing plays a crucial role.
Clinical Diagnostics
In the realm of medicine, clinical diagnostics tops the list of applications for gene sequencing. By analyzing a patient’s genetic makeup, healthcare providers can identify mutations that cause or contribute to diseases. This process leads to more accurate diagnoses and sophisticated treatment options.
For instance, genetic testing can detect hereditary conditions before symptoms arise, allowing for early interventions. This means patients can receive personalized treatment plans tailored to their genetic backgrounds. Moreover, targeted therapies can be developed that address specific mutations, improving patient outcomes. In oncology, for example, sequencing helps identify specific cancer mutations which can indicate the most effective treatment strategies.
"Gene sequencing is revolutionizing how we approach patient care, making precision medicine not just a possibility, but a reality."
Personalized Medicine
Personalized medicine is another significant application of gene sequencing. It is predicated on the idea that each individual's genetic profile can guide the treatment approach. This field leverages gene sequencing data to tailor medications and therapies based on a person's genetic variations.
With advancements in technology, pharmacogenomics has emerged, focusing on how genes affect a person’s response to drugs. For example, patients undergoing treatment for depression may respond differently to certain medications based on their genetic profiles. Through gene sequencing, healthcare providers can ascertain which drugs will be most effective or may cause adverse reactions. This leads not only to improved efficacy but also minimizes the risk of side effects, benefiting patients significantly.
Agricultural Biotechnology
Gene sequencing is not limited to human health; its applications extend profoundly into agricultural biotechnology. In this sector, sequencing plays a pivotal role in crop improvement. By understanding the genetic underpinnings of various crops, researchers can develop strains that are more resilient to disease, pests, and environmental conditions.
Moreover, gene sequencing allows for the monitoring of genetic diversity, essential for sustainable agricultural practices. As climate change poses new challenges, having crops equipped with adaptive traits becomes imperative. For example, gene editing technologies, such as CRISPR, apply sequencing data to make precise alterations in plant genomes, enhancing traits like drought resistance or nutritional content.
Challenges in Gene Sequencing
Gene sequencing is a powerful tool that unlocks the secrets of genetic material. However, the journey through gene sequencing is not without its hurdles. Understanding the challenges in gene sequencing is essential for appreciating the complexity of this field. It highlights technological limitations, data management issues, and ethical considerations. Each of these challenges plays a critical role in shaping the practice and application of sequencing technologies, making them significant topics of discussion.
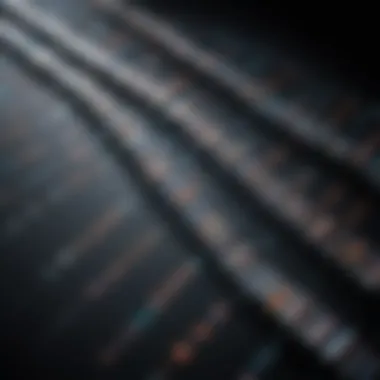
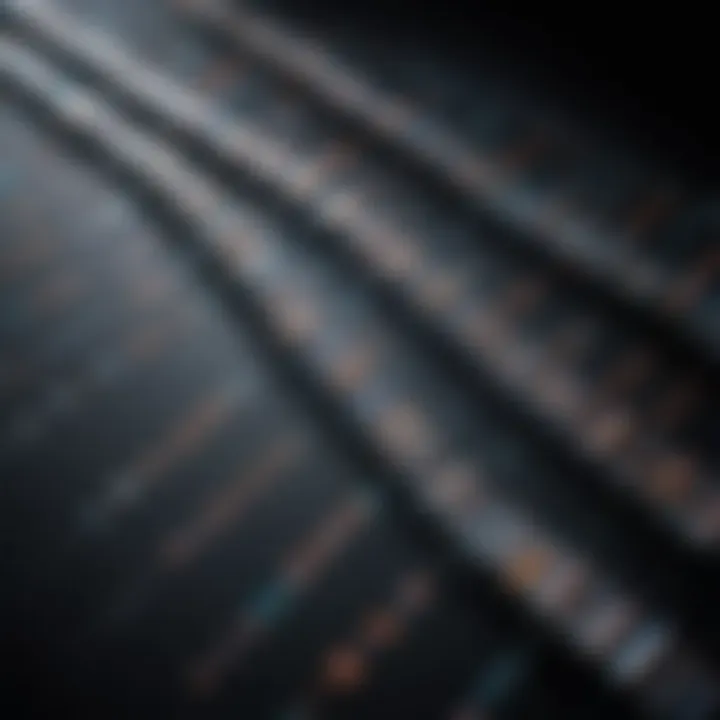
Technological Limitations
Technological limitations are a prominent challenge in gene sequencing. Despite the advancements in sequencing technologies, issues still arise. For instance, not all sequencing platforms deliver the same accuracy or depth of coverage. Some methods can struggle with repetitive regions of DNA or highly similar sequences, leading to gaps in the data.
Moreover, cost remains a significant factor. While costs have decreased over the years, high-quality sequencing can still be prohibitively expensive for many labs. This economic barrier may prevent smaller institutions from engaging fully in cutting-edge genomic research. The need for better technology that is both affordable and accessible is increasingly recognized in the scientific community.
Data Management Issues
Data management is another considerable challenge in gene sequencing. The vast amounts of data generated from sequencing processes require robust systems for storage, analysis, and sharing. With advancements in sequencing technology, the quantity of data has grown exponentially. As a result, the ability to effectively analyze and interpret this data becomes critical.
Many researchers may face difficulties in managing genomic data due to varying formats, standards, and tools. Integrating data from different sources, platforms, and studies poses additional complexity. There is also the challenge of ensuring data security, particularly in clinical settings, where patient information must remain confidential.
Ethical Considerations
Ethical considerations in gene sequencing cannot be overlooked. As we dive deeper into genetic information, questions of privacy, consent, and ownership emerge. The collection of genetic data raises concerns about how this information will be used, who has access to it, and the implications for individuals and populations.
Issues surrounding informed consent are particularly pertinent. Patients must understand the scope of the data being collected, the potential for its future use, and the risks involved. Furthermore, there are concerns regarding the potential for genetic discrimination in employment or insurance based on an individual's genetic predisposition to certain conditions.
"The ethical landscape in gene sequencing continues to evolve alongside technological advancements, necessitating ongoing discussions and guidelines within the field."
In summary, the challenges in gene sequencing extend beyond the laboratory. They encompass technological, data management, and ethical dimensions that are critical for responsible research and application. Understanding these challenges can foster more effective strategies and policies to facilitate the advancement of gene sequencing.
Future Directions in Gene Sequencing
The field of gene sequencing is rapidly evolving, and its future holds significant promise. Understanding the future directions in gene sequencing is crucial as it can open new avenues for research and clinical applications. As technology improves, it can lead to more efficient, cost-effective, and accurate sequencing methods. This can enhance our comprehension of complex genetic diseases and even contribute to breakthroughs in treatment options.
Emerging Technologies
New technologies are continuously reshaping the landscape of gene sequencing. For instance, CRISPR-Cas9 remains a focal point of interest due to its applications in both sequencing and editing genes. This tool allows precise modifications, helping researchers to study genetic variations more effectively.
- Nanopore Sequencing: This technology enables real-time sequencing of DNA. By measuring the changes in electrical current as DNA strands pass through a nanopore, it provides long-read sequencing capabilities. This can uncover complex genomic regions that short-read sequencing might miss.
- Synthetic Biology: Advances in synthetic biology can lead to the creation of new sequencing methods that are not only faster but also more accurate. Researchers are investigating how engineered living systems can contribute to sequencing.
The rise of these technologies may reduce costs and increase accessibility, allowing broader populations to benefit from gene sequencing.
Integration with Other Omics
The integration of gene sequencing with other omics disciplines, such as proteomics and metabolomics, is a promising area for future exploration. This cross-disciplinary approach offers a more comprehensive view of biological systems. By combining data from different omics, researchers can:
- Identify Biomarkers: Integration helps in discovering biomarkers for various diseases, which are essential for diagnosis and treatment.
- Understand Interactions: It sheds light on how genes interact with proteins and metabolites, leading to a holistic view of cellular functions.
- Enhance Personalized Medicine: This integration facilitates the development of personalized treatment plans based on an individual's genetic makeup and metabolic profile.
Integrating data from diverse sources can lead to valuable insights that may otherwise remain hidden when analyzing genomics alone. This is essential for advancing personalized medicine and improving treatment outcomes.
Future advancements in gene sequencing technologies and the integration with other omics can significantly enhance our understanding of genetics, bringing us closer to tailored medical solutions.
End
The conclusion serves as an integral part of this article, synthesizing the various threads presented throughout the exploration of gene sequencing. It encapsulates the critical insights gained, discussing how the evolution of sequencing methods has opened new avenues in genetic research and applied sciences. Through a thorough examination of the technologies, processes, and applications discussed, the conclusion highlights the interconnectedness of these elements, framing them within the larger narrative of scientific progress.
Recap of Key Points
In summary, several key points demand reiteration to emphasize their significance:
- Technological Evolution: The transition from Sanger sequencing to next-generation and third-generation sequencing technologies signifies advancement in efficiency, accuracy, and throughput in genetic studies.
- Process Understanding: A comprehensive grasp of each step—from sample preparation to data analysis—is crucial. Each stage directly influences the quality of final genetic data.
- Wide Applications: Gene sequencing plays a pivotal role in various fields such as clinical diagnostics, personalized medicine, and agricultural biotechnology, demonstrating its versatility and impact.
- Emerging Challenges: As sequencing becomes more widespread, challenges such as data management and ethical considerations must not be overlooked. Societal implications need thoughtful discussion as the technologies evolve.
The Importance of Continued Research
Continued research in gene sequencing is vital for several reasons. Innovation in sequencing technologies not only improves existing methods but also introduces new capabilities that could revolutionize understanding of genetics. Researchers must remain committed to exploring the vast potential of gene sequencing.
- Adapting to Technological Changes: As advancements occur, staying abreast of new methodologies ensures that scientific inquiry remains relevant and impactful.
- Addressing Ethical and Social Concerns: Ongoing investigation enables the scientific community to proactively engage with ethical dilemmas presented by genetic data collection and use. Keeping public discourse transparent establishes trust and helps navigate sensitive issues.
- Enhancing Clinical and Research Outcomes: Improved sequencing techniques can lead to enhanced diagnostic tools and more effective treatments, ultimately contributing to advancements in healthcare.
Research efforts, therefore, must prioritize innovation and ethical considerations alike, ensuring that the future of gene sequencing remains robust and responsible. Advancements in this field hold the promise of profound implications for both science and society.
Research in this domain must not waver, as the pursuit of knowledge is an unending journey that shapes our understanding of life itself.
As we move forward, engaging with these aspects ensures that gene sequencing continues to evolve as a cornerstone of genetic research.