Techniques for Gene Insertion Using CRISPR

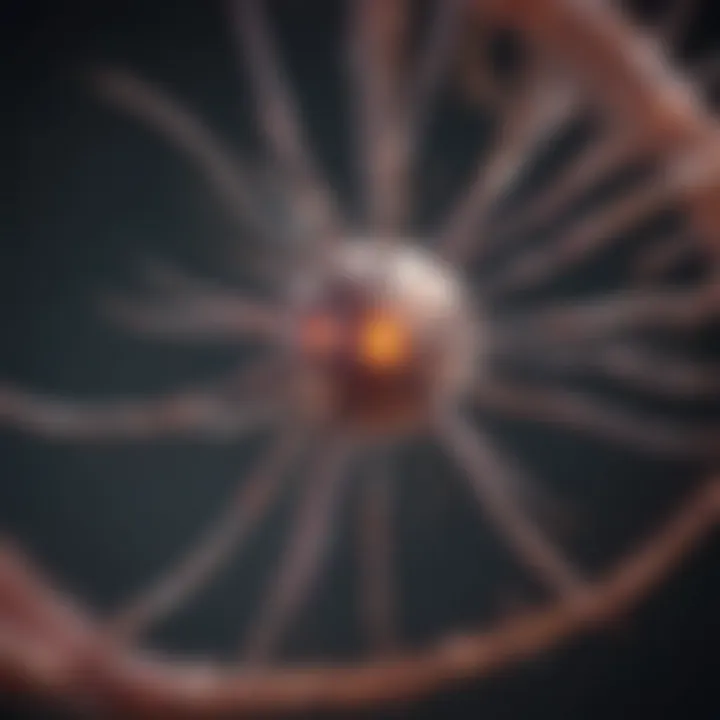
Intro
The advance of gene editing technologies marks a significant milestone in biological research and biotechnology. Among these, CRISPR has emerged as a game changer, offering a precise and efficient method for gene insertion. This technology harnesses a natural defense mechanism found in bacteria, allowing scientists to modify genomes with remarkable accuracy. Within this article, we will delve into the techniques involved in gene insertion using CRISPR, dissecting the various methods and their implications across multiple fields.
As researchers explore the frontiers of genetic engineering, it becomes crucial to understand the intricate workings of CRISPR and its practical applications in gene insertion. Not only does this comprehension pave the way for revolutionary treatments and agricultural enhancements, but it also sparks important discussions around ethical considerations and the future trajectory of genetic manipulation. Intrigued? Let’s embark on a comprehensive exploration of how CRISPR technologies reshape our understanding of genetic engineering!
Foreword to CRISPR Technology
In the evolution of genetic engineering, CRISPR technology stands out as a monumental breakthrough, a true game-changer in the field. Not merely a complex tool for gene editing, CRISPR offers a precision rarely seen in the history of biotechnology. As researchers, educators, and professionals delve into this fascinating realm, understanding CRISPR's foundational elements becomes crucial. This familiarity fosters not only skilled application but also a nuanced comprehension of its implications across various fields.
Overview of CRISPR
The acronym CRISPR, which stands for Clustered Regularly Interspaced Short Palindromic Repeats, represents a fascinating system derived from the immune response of certain bacteria. When a virus invades a bacterium, the bacterium captures snippets of the virus's DNA and integrates them into its own genome, storing them for future defense. This cleverly orchestrated mechanism not only protects the bacterium from subsequent attacks but also provides a template for the development of innovative gene-editing techniques.
The beauty of CRISPR lies not just in its biological origin but in its adaptability. Researchers can harness this natural process, modifying the components—namely, the Cas9 protein and guide RNA (gRNA)—to facilitate gene editing in various organisms. This adaptability opens the door to unprecedented possibilities in medicine, agriculture, and beyond, making CRISPR an essential topic for any serious discussion on the future of genetics.
Historical Development
The journey of CRISPR technology is more than a tale of discovery; it is a reflection of how scientific inquiries evolve through collaborative efforts. Although the CRISPR mechanism was first described in the early 1980s, it wasn't until the early 2000s that a diverse team of scientists began piecing together its potential in gene editing.
Pioneering studies in 2005 by Francisco Mojica shed light on the unique properties of CRISPR sequences and their role in microbial immunity. It was not until 2012 that Jennifer Doudna and Emmanuelle Charpentier engineered CRISPR-Cas9 as a genetic tool, a pivotal moment that triggered a wave of innovation in gene editing. Their groundbreaking work not only earned them the prestigious Breakthrough Prize in Life Sciences but also laid the groundwork for the surging interest in genetic manipulation technologies.
"CRISPR has democratized gene editing, making what was once the domain of well-funded laboratories accessible to smaller research outfits and even educational institutions."
As researchers from various disciplines engaged with CRISPR technology, they began utilizing it to edit genes in an array of organisms, thus expanding its potential applications. The innovation sparked intense discussions about ethical considerations, regulatory implications, and future directions for research in genetics. Such conversations are crucial as scientists navigate the delicate balance between innovation and responsibility, paving the way for the next generation of genetic research.
Understanding the historical development not only casts light on technological advancements but also on the potential this technology holds for the future. Those wishing to dive deeper into the intricacies of CRISPR can consult resources like Britannica for comprehensive insights.
Mechanism of Gene Editing with CRISPR
The mechanism of gene editing through CRISPR technology serves as the backbone of its effectiveness and versatility. Understanding this mechanism is essential because it lays the groundwork for the specific techniques employed in gene insertion. It reveals not only the sophistication of CRISPR but also its potential limitations and challenges. By exploring the components and functions involved, one can appreciate how CRISPR operates at a molecular level, guiding researchers in optimizing these techniques for various applications.
Components of CRISPR
The components of CRISPR play a pivotal role in its functionality. They include the Cas9 protein, guide RNA, and donor DNA, each serving a unique purpose.
Cas9 Protein
The Cas9 protein is an enzyme that plays a crucial role in DNA targeting and cleavage. It acts like a molecular scissors, cutting DNA at specific sites as directed by the guide RNA. This key characteristic makes Cas9 a valuable asset in gene editing. What sets Cas9 apart is its ability to introduce double-strand breaks in the target DNA, allowing subsequent repair mechanisms to either knock out a gene or insert new genetic material.
One unique feature of Cas9 is its dual cut capability, which enhances precision. However, its limitation lies in the potential for off-target effects, where unintended DNA sequences may be altered. Nevertheless, when it comes to specificity and efficiency, Cas9 remains a popular choice in the realm of CRISPR.
Guide RNA
The guide RNA (gRNA) is an essential component that directs the Cas9 protein to the corresponding DNA target. It consists of two parts: a short sequence that is complementary to the target DNA and a scaffold sequence that binds to the Cas9 protein. The major benefit of gRNA is its tailorability; scientists can synthesize gRNAs to target specific genes, making it a flexible tool in gene editing endeavors.
A key characteristic of gRNA is its adaptability, allowing researchers to create custom gRNAs for various targets. This feature contributes significantly to the precision of CRISPR technology. On the flip side, designing gRNAs that are specific enough to avoid off-targeting remains a challenge, necessitating careful validation.
Donor DNA
The donor DNA serves as the template for the insertion of new genetic material. This element is crucial when attempting to insert or replace specific genes. A key advantage of donor DNA is its capacity to carry the desired genetic sequences that guide the cellular repair processes. The design of donor DNA can drastically influence the success of gene insertion, rallying it as a focal point in CRISPR applications.
A notable feature of donor DNA is that researchers can engineer it to include additional elements, such as promoters or other regulatory sequences. However, disparities in how different cells utilize donor DNA during repair can yield inconsistent results. This inconsistency represents a challenge that scientists continue to navigate in their gene editing pursuits.
How CRISPR Functions
CRISPR's effectiveness hinges upon its methodical operation, which can be broken down into three essential phases: target site recognition, DNA cleavage, and subsequent repair mechanisms.
Target Site Recognition
Target site recognition is the initial step where the guide RNA pairs with the complementary DNA sequence in the genome. This specificity is vital for ensuring that Cas9 initiates a cut at the precise locus intended. The ability of gRNA to recognize specific sequences paired with the precision of the Cas9 protein presents a significant edge in gene editing.
One of the advantages of target site recognition is its efficiency; a well-designed gRNA significantly increases the likelihood of successful targeting. However, due to the complexity of genomes, designing gRNAs remains a delicate balance between specificity and activity, often presenting challenges in terms of achieving optimal outcomes.
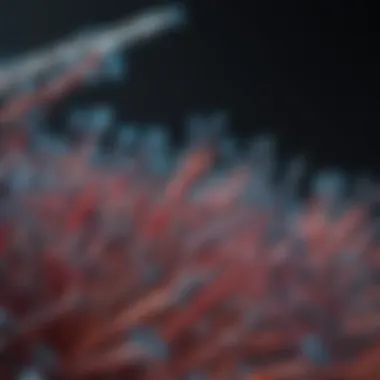
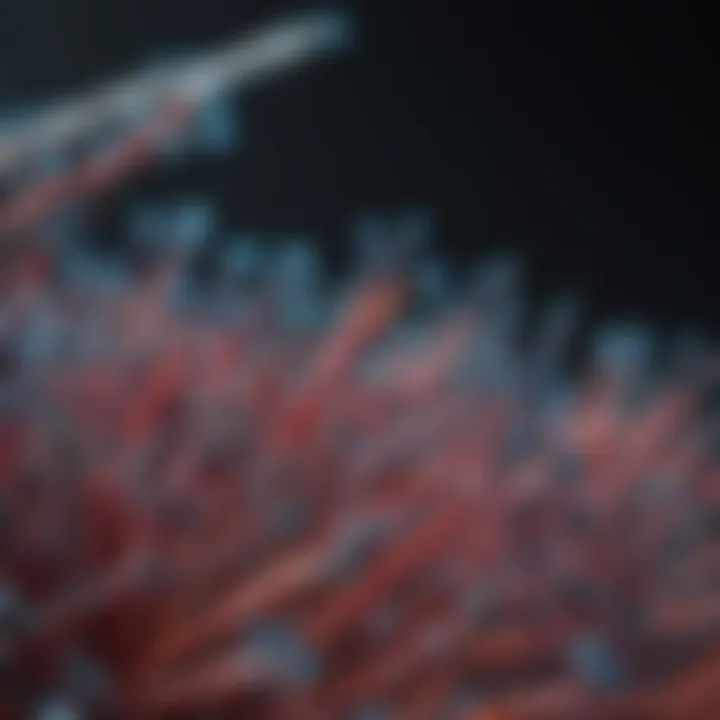
DNA Cleavage
After successful recognition, the Cas9 protein performs DNA cleavage, creating a double-strand break. This cleavage is a critical juncture in the CRISPR process, as it sets the stage for the subsequent gene editing strategy. The precise cutting mechanism of Cas9 at the target site is one of its most significant strengths, facilitating targeted intervention into genomic sequences.
Despite this precision, the potential for off-target cuts cannot be understated. If Cas9 mistakenly cleaves unintended DNA sequences, it can induce unwanted genetic alterations, raising concerns about safety and efficacy in clinical applications.
Repair Mechanisms
Once DNA is cleaved, the cell's natural repair mechanisms come into play to mend the break. Two primary pathways exist: non-homologous end joining (NHEJ) and homology-directed repair (HDR). Each pathway serves distinct purposes in gene editing. NHEJ tends to lead to insertions or deletions, effectively knocking out genes, while HDR allows for precise gene insertion from donor DNA. The selection of the repair mechanism can significantly influence the outcome of gene editing.
While HDR is favorable for precise modifications, it is often less efficient, especially in the context of certain cell types or tissue backgrounds. Consequently, researchers must scrutinize the mechanism's efficiencies and the desired outcomes to optimize the CRISPR applications.
In summary, a nuanced understanding of the mechanism of gene editing with CRISPR offers insights into its applications and potential obstacles, informing better strategies for gene insertion.
Techniques for Gene Insertion
The process of gene insertion through CRISPR technology represents a significant advancement in genetic engineering. This topic is pivotal not just for its technical implications but also for the breadth of applications it supports. By using specific methods tailored for gene insertion, researchers can optimize the precision and efficiency of their experiments, which has transformative potential across fields like agriculture, medicine, and biological research. The ability to insert genes accurately can lead to enhanced crop varieties, novel therapeutic options, and deeper biological insights. Understanding these techniques can directly relate to improved outcomes in research and practical applications.
Vector-Based Methods
Vector-based methods are foundational in gene insertion, relying on vehicles that can carry genetic material into target cells. These vectors can either be derived from viruses or constructed as plasmids, each with specific attributes that render them effective in their roles.
Viral Vectors
When it comes to viral vectors, their primary allure lies in their remarkable efficiency at delivering genes into a host's genome. Viruses, by nature, have evolved to penetrate cells, making them adept at gene transfer. This characteristic grants viral vectors the edge when researchers aim to achieve a high rate of gene integration. However, one must also consider the specificity of the viral type used, as different viruses can exhibit varying effects on different cell types.
A notable feature of viral vectors is their potential for stable expression of the inserted genes, which is particularly beneficial in long-term studies. However, the use of viral vectors is not without its disadvantages. For instance, there’s a risk of inducing an immune response or unwanted mutations. This complexity makes choosing the right viral vector a balancing act between efficacy and safety.
Plasmid Vectors
On the other hand, plasmid vectors represent a more straightforward approach to gene insertion, particularly valued for their ease of use. Plasmids are small, circular DNA molecules that replicate independently within cells. A key characteristic of plasmids is their ability to be engineered rapidly and cultivated in large quantities, allowing for a flexible approach to gene insertion.
Plasmids can also facilitate the co-existence of multiple genetic sequences within a single context, making them a versatile choice for certain experimental designs. However, they may lack the efficiency of viral vectors in some situations, especially in difficult-to-transfect cell types. Their transient expression often leads to the challenge of maintaining gene activity over longer periods, making them less suitable for certain applications.
Preassembled RNPs
Preassembled ribonucleoprotein (RNPs) systems offer a cutting-edge approach for gene insertion that combines both efficiency and precision. This technique involves assembling the key components—Cas9 and guide RNA—outside the cell before introducing them together into the target organism. This ensures that the editing tools are correctly aligned and ready immediately upon entry.
Mechanism of RNP Insertion
The mechanism of RNP insertion draws from the natural function of CRISPR. Here, the Cas9 enzyme comes into play, recognizing and binding to the target DNA sequence guided by the RNA. This setup allows for highly specific editing without the extensive preparation required by other methods. The uniqueness of RNPs lies in their ability to reduce off-target effects since the components are delivered as a ready-to-go entity.
The precision of RNPs is a notable advantage, but one must consider factors such as the cell type and delivery method, which can influence the success rate.
Advantages of RNPs
The advantages of using RNPs are particularly compelling, especially for those aiming at minimal off-target effects. The short-half life of the RNPs can also be beneficial in specific contexts, helping ensure that the editing tools are not lingering longer than necessary in the cellular environment. This immediacy can reduce immune reactions and enhance the safety profile when used in therapeutic applications.
Nevertheless, the complexity in preparing RNPs and their relatively high cost can be barriers to their widespread use compared to more traditional methods.
Electroporation Techniques
Electroporation has emerged as another powerful method for gene insertion, leveraging electrical fields to enhance cell membrane permeability. This allows for the effective introduction of CRISPR components directly into various cell types, broadening the spectrum of applications.
Principles of Electroporation
The principles behind electroporation are relatively straightforward: applying short bursts of electrical pulses to cells transiently opens pores in the cellular membrane. Once these temporary openings are created, CRISPR components can flow into cells more freely. One of the standout features of this technique is its capability to be applied to a wide range of cells, from tough plant cells to delicate mammalian cells.
While the efficacy of electroporation is promising, it is essential to fine-tune the electrical parameters. Selecting the right voltage and pulse duration can affect cell health and viability, which makes rigorous optimization crucial.
Applications and Case Studies
Electroporation techniques have found diverse applications, particularly in fields like gene therapy and plant biotechnology. For instance, in studies focused on improving crop resilience, researchers utilize electroporation to facilitate the introduction of CRISPR systems directly into plant protoplasts, streamlining the genetic editing process. Similarly, in clinical research, electroporation has enabled the delivery of therapeutic genes in oncology, showcasing its potential across different biological systems.
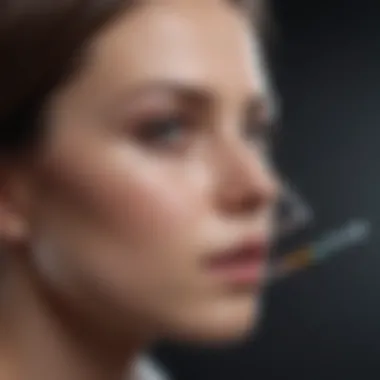
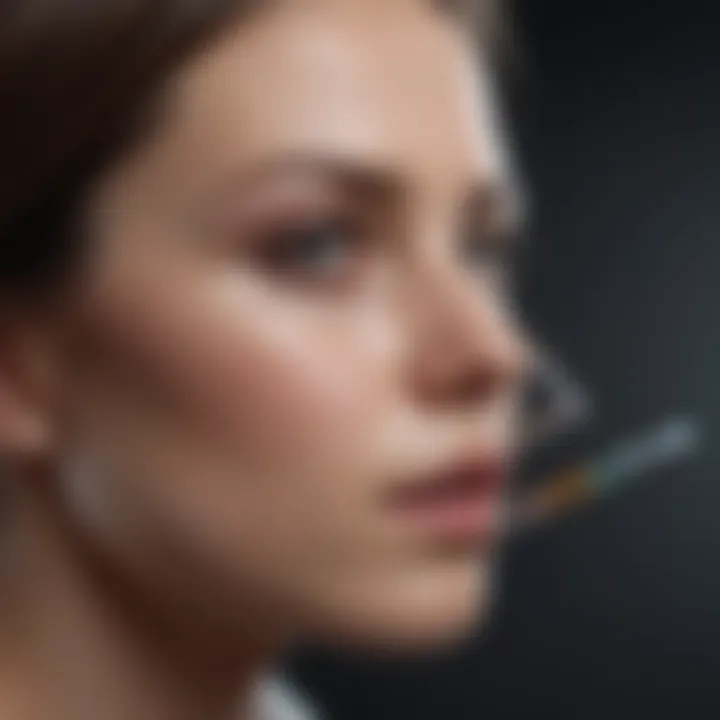
Though effective, electroporation’s applicability can sometimes be limited by the type of cells being used, necessitating further exploration to enhance its effectiveness across varied contexts.
Applications of CRISPR Gene Insertion
The advent of CRISPR technology has opened up a veritable Pandora’s box of possibilities in the realm of gene insertion. It’s not just a scientific curiosity—this technology is reshaping various industries by allowing precise alterations in genetic makeup. The ability to edit genes on demand serves as a game-changer, enabling researchers to not only understand but also manipulate genetic material in a controlled manner. This section provides an in-depth look at the multifaceted applications of CRISPR, particularly in agriculture, medicine, and basic research.
In Agriculture
CRISPR gene insertion is making waves in agriculture, where improving crops is more than just an exercise in patience; it’s a necessity. Traditional breeding methods have their merits, but they often fall short of meeting the pressing demands of the growing population. With multifactorial challenges, such as climate change, pests, and diseases, CRISPR is emerging as a knight in shining armor.
- Increased Yield: One of the standout benefits is the potential for increased crop yield. By targeting genes associated with growth regulation, scientists can create strains of crops that are more productive. This opens doors for food security, particularly in regions facing scarcity.
- Disease Resistance: CRISPR technology allows researchers to insert genes that can enhance plants’ resistance to diseases, reducing the reliance on chemical pesticides. This shift not only has economic implications but also benefits the environment.
- Nutritional Enhancement: Researchers are working on inserting genes that boost the nutritional content of staple crops. For example, rice enriched with vitamins could have a significant impact on public health in areas prone to nutrient deficiencies.
Yet, this innovation is not without hurdles. Regulatory frameworks are catching up with scientific advancements. There’s an ongoing dialogue about the broader implications of gene-edited crops, which leads us to consider the social acceptance of CRISPR-driven modifications.
In Medicine
When it comes to healthcare, the CRISPR technique is like holding a scalpel to a complex malady. It opens the door to targeted therapies that were once just ideals on a drawing board. The applications in medicine are profound, from treating genetic disorders to developing advanced therapies for cancer.
- Gene Therapy: Here, CRISPR allows for direct modification of genes responsible for inherited disorders. For instance, disorders like cystic fibrosis and sickle cell disease might one day be treated by simply correcting the faulty genes.
- Personalized Medicine: By analyzing individual genetic profiles, CRISPR can facilitate the creation of personalized treatment plans tailored to a patient’s specific genetic makeup, marking a step toward individualized healthcare.
- Vaccine Development: Researchers are also exploring CRISPR’s role in creating new vaccines, particularly in light of global health crises. The technology can speed up the development process while maintaining precision, which is critical in tackling emerging infectious diseases.
Nevertheless, ethical discussions surrounding gene editing in humans are crucial. Questions arise about the ramifications of ‘playing God’ and what it means for future generations.
In Basic Research
Basic research stands as the bedrock of scientific advancement, and CRISPR technology is enhancing our ability to explore the fundamental mysteries of biology. This tool is reshaping research methodologies, allowing scientists to investigate gene functions with unprecedented precision.
- Functional Genomics: With CRISPR, researchers can create gene knockouts to study the effects of gene removal on organismal health and development. It paves the way for a deeper understanding of gene interactions and biological pathways.
- Model Organisms: By generating genetically edited organisms, scientists can better simulate human diseases in model organisms like mice or zebrafish. This leads to breakthroughs in drug discovery and disease understanding.
- Synthetic Biology: CRISPR is instrumental in synthetic biology, where researchers can engineer microbes to produce valuable compounds or biofuels, contributing to sustainable practices.
The future of basic research using CRISPR is promising but demands a careful balance between innovation and ethical considerations. As we explore the depth of genetic materials, it's imperative to maintain a conscientious approach to scientific inquiry.
"The implications of CRISPR's applications are vast, yet they invite scrutiny that is essential for responsible science."
In summary, the applications of CRISPR gene insertion are as expansive as the horizon before a scientist. From agriculture to healthcare to research, each element underscores the potential benefits and ethical challenges associated with genetic editing. As awareness grows, so does the conversation around responsible use, highlighting the inherent responsibility that comes with such power.
Ethical Considerations in Gene Insertion
In the realm of gene editing, particularly using CRISPR technology, ethical considerations loom large. This topic is important because it doesn’t just touch on scientific innovation; it also probes the moral, social, and regulatory aspects that arise from the manipulation of genetic material. Gene insertion via CRISPR opens doors to many possibilities, but with such power comes great responsibility. Understanding the ethical implications is essential for researchers and practitioners in this field, as well as for the general public, who might be affected by these advancements.
When discussing ethical considerations, it’s crucial to weigh the potential advantages against the possible risks and societal impacts. These factors can guide the practices and policies governing CRISPR usage, ensuring that the technology is harnessed for the greater good, without crossing ethical boundaries.
Regulatory Frameworks
Regulatory frameworks surrounding gene editing technologies like CRISPR are key to balancing innovation with safety and ethical integrity. Different countries approach gene insertion regulations with varying degrees of stringency. Some places have established comprehensive guidelines, whereas others are still grappling with how to manage this powerful tool.
Key points regarding regulatory frameworks include:
- Approval Processes: Before any clinical trials involving human subjects, thorough evaluations by regulatory bodies such as the Food and Drug Administration (FDA) in the United States ensure that the implementation of CRISPR applications is safe and effective.
- Biosafety Standards: Regulations enforce biosafety measures to prevent unintended releases of genetically modified organisms into natural ecosystems, which could have unforeseen ecological consequences.
- Public Engagement: Some frameworks emphasize the importance of public consultation, allowing communities to express their concerns and insights regarding gene editing practices. This step is pivotal in addressing public fears and expectations, not to mention fostering trust between scientists and the populace.
The regulatory landscape is constantly evolving, as new discoveries emerge and public sentiment shifts. This adaptability is essential to ensure that ethical standards keep pace with scientific advancements.
Moral Implications
The moral implications of gene insertion can't be overstated. At the core of the conversation is the question of what it means to manipulate life at such a fundamental level. Proponents argue that CRISPR can lead to groundbreaking medical advancements, such as eradicating genetic diseases and improving agricultural resilience. However, critics warn against potential misuse and the moral pitfalls of 'playing God'.
Some significant moral considerations include:
- Equity and Access: As gene editing technology becomes more prevalent, concerns rise about who gets access to these advancements. Is it just the wealthy that benefit, or can equitable frameworks be established?
- Genetic Discrimination: There is a fear that gene editing could lead to a new form of discrimination, where individuals are judged or treated differently based on their genetic modifications.
- Long-term Effects: There are uncertainties about the long-term implications of gene editing. Changes made today could have cascades of unforeseen effects on future generations, raising questions about the responsibility researchers hold over their innovations.
In summary, the ethical considerations surrounding CRISPR gene insertion emphasize not only the scientific potential but also the societal responsibilities that come with it. Engaging with these ethical dimensions is key for anyone involved in genetic engineering, ensuring that science and morality walk hand in hand as we forge ahead into this exciting new frontier.
Challenges in CRISPR Gene Insertion
Gene insertion using CRISPR technology presents not only remarkable opportunities but also significant challenges that researchers must navigate. These challenges are intricate and can dictate the success or failure of an experiment. Recognizing the nuances involved is essential for scientists aiming to advance genetic engineering effectively.
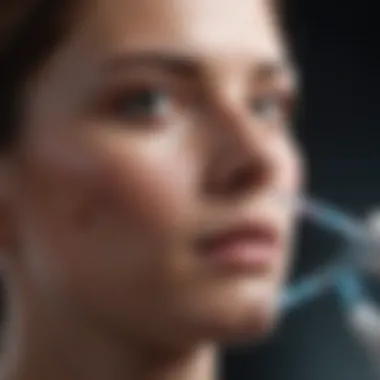
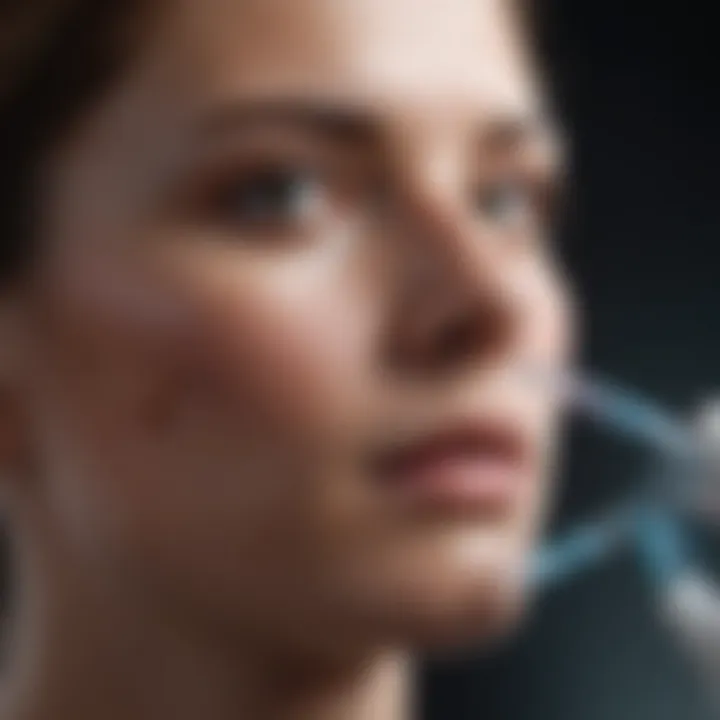
It's important to address these challenges because they not only inform the design of better experiments but also establish a framework for ethical considerations in genomic research. Failures in gene insertion can have far-reaching implications, impacting everything from agricultural advancements to medical therapies. Thus, understanding these obstacles is crucial for any comprehensive exploration of CRISPR methods.
Off-Target Effects
One of the most pressing challenges in CRISPR technology is off-target effects, where the editing process inadvertently alters sequences in unintended regions of the genome. This misstep arises primarily because the guide RNA, which is supposed to direct the Cas9 protein to the desired target, has a chance of binding to similar, but not identical sequences elsewhere in the genome. The ramifications can be serious: unintended mutations could lead to errors that may produce harmful effects, such as the activation of oncogenes or the silencing of essential genes.
- Potential Risks: Off-target alterations are not merely theoretical but have been documented across various studies. A well-known 2016 paper highlighted that nearly 10% of the attempts resulted in off-target mutations. This means that genetic integrity could be compromised, increasing the risk of adverse effects in therapeutic applications.
- Specificity Improvement: Scientists are continuously working on improving the specificity of CRISPR systems. Variants like High-Fidelity Cas9 and eSpCas9 have been engineered to minimize these off-target events. Moreover, utilizing paired gRNAs can increase precision, as dual targeting is less forgiving of mismatched sequences.
Ultimately, while the promise of CRISPR gene editing is immense, off-target effects remain a critical concern that requires continued innovation and awareness.
Delivery Mechanism Issues
An even more fundamental challenge lies in the delivery mechanisms used to introduce CRISPR components into target cells. The efficacy of gene insertion heavily relies on how well these components can reach their destination within a cell. Different delivery techniques carry their own sets of limitations, leading to varying degrees of success.
- Common Methods: The main delivery strategies include electroporation, viral vectors, and lipid nanoparticles. Each method comes with its pros and cons. For example, while viral vectors offer high efficiency, they pose risks of insertional mutagenesis.
- Selection of Delivery Systems: The choice of method often boils down to the type of cells being targeted. For example, hard-to-transfect cells like primary neurons may necessitate more sophisticated methods, such as using custom nanoparticles.
- Retargeting Dynamics: Moreover, understanding the retargeting of gene editing components within a cellular environment is essential. The journey from the extracellular space into the nucleus involves barriers that may impede the success of gene insertion. Overcoming these barriers varies not just by delivery method but often by the nature of the tissue being targeted.
"The success in CRISPR applications hinges not only on the accuracy of gene editing but also on the delivery methodologies employed to reach the heart of the genetic material."
In summary, the challenges posed by off-target effects and delivery mechanisms are not mere obstacles but rather opportunities for improvement. As researchers continue to tackle these issues, the future of CRISPR technology can only get brighter.
Future Directions in CRISPR Research
As we stand at the precipice of a biotechnological renaissance, the future of CRISPR research reveals itself not merely as a continuation of existing practices, but as a dynamic landscape ripe with new potential. Understanding where the road will lead us in the application of CRISPR technology is paramount for several reasons, chiefly among them being the necessity to address ongoing challenges while effectively harnessing the capabilities it offers. The crux here lies in refining and expanding gene editing techniques to embrace innovative solutions for a myriad of complex problems faced in medicine, agriculture, and beyond.
With advancements in precision and efficacy, next-generation methodologies are key to ensuring that CRISPR can consistently deliver on its promises without the shackles of vulnerabilities. As researchers delve deeper, they will unearth novel techniques that not only enhance accuracy but also broaden the horizons of what gene editing can achieve.
"The essence of scientific progress rests on the shoulders of those who dare to explore beyond the known."
Novel Techniques
The innovative path ahead intertwines with several groundbreaking techniques aimed at improving CRISPR efficacy and reducing potential risks. One area gaining traction is the development of base-editing technology, which allows for precise changes to the sequence of DNA without making double-strand breaks. This eliminates some risks related to traditional CRISPR-Cas9 methods, such as unwanted mutations at off-target sites.
Another promising avenue is the advent of prime editing. Described as a "search-and-replace" method for DNA, prime editing has the potential to make the editing process much more versatile. It can target specific locations within the genome and enables more complex alterations without the same level of irreversibility that previous techniques presented.
Furthermore, enhancements in delivery systems—like lipid nanoparticles—open the gates for more effective and less invasive approaches to gene insertion. These novel delivery methods help tackle challenges associated with bioavailability, ensuring that CRISPR components reach their designated target efficiently and with minimal degradation.
- Base Editing - A precise method that modifies DNA bases without disrupting the backbone.
- Prime Editing - Allows for more complex edits to be conducted with greater precision.
- Enhanced Delivery Systems – Techniques to improve the introduction of CRISPR components to target cells.
Expanding Applications
The reach of CRISPR stretches far and wide, and as research progresses, the applications of this technology are set to explode in diversity. From agricultural advancements to medical breakthroughs, the implications are profound.
In the realm of agriculture, CRISPR's capacity to create crops that can withstand pests or environmental stressors more efficiently can reshape food security. The importance of genetically edited crops in combating climate change cannot be understated. Furthermore, efforts to engineer plants that can produce higher yields without chemical fertilizers are on the rise.
Additionally, the therapeutic potential of CRISPR in medicine is phenomenal. Real-world applications like editing genes for rare genetic disorders, enhancing immune responses for cancer treatment, and developing personalized medicine strategies are steadily aligning more with clinical practice. This dynamism opens doors for future innovations that can potentially eradicate diseases that currently have no cure.
Overall, the ongoing exploration into the future of CRISPR entails a blend of responsibility, creativity, and ambition, setting the stage for significant scientific advancements and societal benefits.
Culmination
As we bring our exploration of gene insertion techniques to a close, it’s essential to recognize the significance of this discussion in the broader context of CRISPR technology. Understanding these methods not only illuminates the current capabilities of genetic engineering but also highlights future potentialities in various fields.
Summary of Key Points
In summarizing the key points, we can identify several crucial aspects related to CRISPR gene insertion:
- Diverse Techniques: From vector-based methods such as viral and plasmid vectors to innovative strategies like Preassembled RNPs and electroporation, each technique presents unique advantages and limitations to be considered.
- Applications Across Fields: The versatility of gene insertion techniques has shown significant promise in agriculture, medicine, and basic research. For example, in crops, CRISPR can enhance yield or resistance to pests. In medicine, gene editing may pave the way for treatments of genetic disorders.
- Ethical Considerations: While exciting, these advancements come with complex moral implications and the need for robust regulatory frameworks, ensuring responsible usage of this powerful tool.
- Challenges Faced: There are concerns regarding off-target effects and difficulties in delivery mechanisms which researchers must navigate to achieve reliable results in gene insertion.
Each of these factors contributes to a nuanced understanding of CRISPR's landscape and the careful consideration needed in applying these powerful techniques within the real world.
Final Remarks on CRISPR Technology
In reflecting on CRISPR technology, it’s clear that we stand at the cusp of revolutionary advancements. The capabilities of gene insertion illustrate the remarkable potential this technology holds. The ability to edit genes opens doors to solving pressing challenges in health and agricultural productivity.
However, with power comes responsibility. As researchers and practitioners, it’s vital to approach CRISPR applications with caution and integrity. Continuous dialogue and collaboration among scientists, ethicists, and regulators are necessary to navigate the landscape with care. The path ahead is promising, yet laden with responsibilities that will shape the future of genetic engineering.
"Gene editing is not just a tool; it’s a key to unlocking the puzzle of life itself."
For anyone eager to deepen their knowledge or pursue research in this area, keep an eye on evolving methodologies and practical applications stemming from ongoing studies. The journey into genetic modification is just beginning.