Innovations in Plasma Electricity Generation
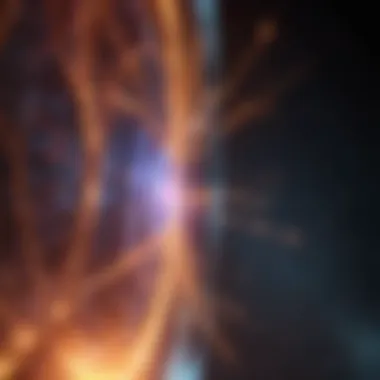
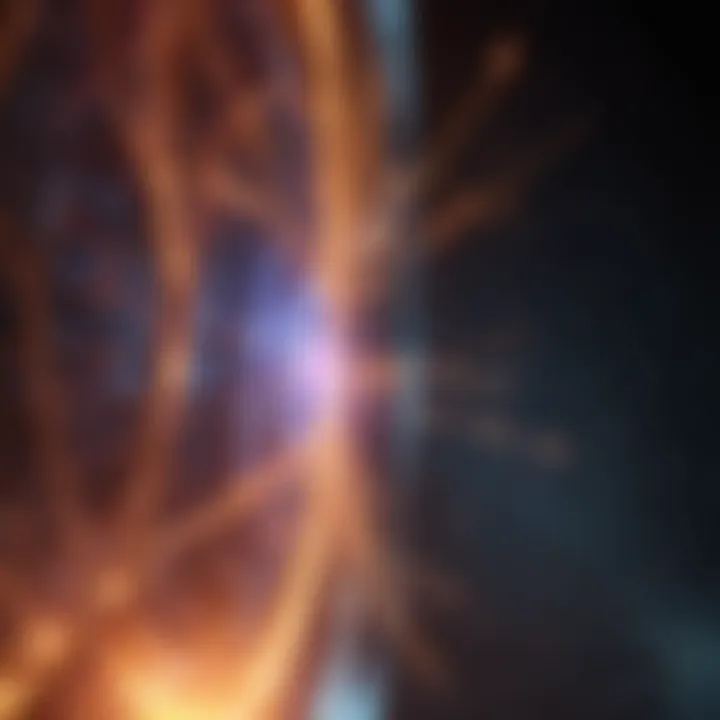
Intro
Plasma electricity generation is a captivating area of study that bridges the gap between physics and energy technology. Its significance is becoming ever more pronounced as the global demand for alternative energy sources increases. In simple terms, plasma is an ionized gas, which means it contains charged particles—electrons and ions. This unique state of matter allows for innovative methods of generating electricity that are not only efficient but also have the potential to revolutionize energy production.
This article aims to dissect the intricate principles governing plasma electricity generation, while also highlighting the latest innovations making waves in the field. The evolution of plasma technology could possibly hold the key to solving many of today's energy challenges. By examining methods, applications, and future directions, we hope to provide a thorough understanding suitable for students, researchers, or professionals entering this dynamic area of science.
Research Overview
Summary of Key Findings
The preliminary investigations into plasma generation mechanisms have unveiled some fascinating insights:
- Variety of Generation Techniques: Plasma can be produced through several methods including radio frequency, lasers, and microwave discharges. Each technique carries its own set of advantages and challenges.
- High Efficiency: Innovations in plasma technology have demonstrated energy output that rivals traditional methods, making it an attractive alternative for sustainable energy.
- Environmental Implications: The shift towards plasma electricity generation may lead to lower carbon emissions compared to conventional fossil fuel sources.
- Adaptability: Plasma systems show promise for various applications, from energy production to waste management and material processing.
Significance of Research
The relevance of this research cannot be overstated. Energy production is at a crossroads; higher efficiency, reduced environmental impact, and sustainable practices are imperative for future economies. By understanding the principles of plasma physics and exploring practical applications, stakeholders can harness these advantages. For instance, researchers and industry professionals might find new pathways for advancement in clean energy technology, ultimately contributing towards energy security and sustainability.
Methodology
Research Design
To thoroughly investigate plasma electricity generation, a mixed-method approach is often utilized. This includes both qualitative and quantitative research methodologies to ensure a robust exploration of the topic.
Data Collection Methods
Data for this research can be collected through:
- Literature Review: Compiling existing studies and papers to understand the theoretical foundations and current state of the field.
- Experimental Analysis: Conducting practical experiments to measure plasma generation efficiency under different conditions.
- Interviews with Experts: Engaging with professionals to gain insights into recent innovations and industry challenges.
"The leap toward plasma-based systems represents not just a technological shift, but also a transformative approach to how we think about energy."
In summary, this exploration into plasma electricity generation aims to intersect theoretical and practical knowledge, challenging existing paradigms in energy production while setting the stage for future developments.
Prologue to Plasma Electricity Generation
Plasma electricity generation stands at the crossroads of technology and science, offering promising pathways for the future of energy production. As power requirements escalate globally, the quest for cleaner and more efficient energy sources intensifies. Plasma, the fourth state of matter, presents a unique opportunity to explore innovative generation methods that could transform energy systems. Understanding the principles and applications behind plasma generation is not just an academic exercise; it's fundamental for developing sustainable energy solutions.
Definition and Relevance
Plasma can be defined as an ionized gas, consisting of charged particles, including ions and electrons. Unlike solids, liquids, or gases, plasma behaves distinctly due to its electric and magnetic field interactions. In the context of electricity generation, plasma plays an essential role. It can produce energy through various methods, such as nuclear fusion, and can significantly enhance combustion processes in engines. The relevance of plasma electricity generation is amplified by the drive to reduce carbon emissions and reliance on fossil fuels.
The benefits include:
- High efficiency: Plasma systems can convert energy with impressive efficiency rates compared to conventional energy methods.
- Environmental advantages: Many plasma generation techniques promise lower emissions and can utilize waste materials.
- Versatility: Plasma technologies find applications not just in energy, but also in aerospace, healthcare, and materials science.
Historical Context
The journey of plasma generation dates back to the early 20th century. The distinct characteristics of plasma began to be studied in earnest after the discovery of radioactivity. Scientists like Irving Langmuir and Michael Faraday contributed foundational knowledge regarding ionization and discharge phenomena. In the post-World War II era, with advancements in nuclear technology, plasma research shifted gears, gaining traction in fusion energy projects. Early fusion experiments, such as Project Orion, paved the way for contemporary initiatives aimed at harnessing plasma for practical energy solutions.
As the decades rolled on, notable milestones emerged, including:
- Development of the tokamak design for nuclear fusion reactors.
- Utilization of plasma technologies in semiconductor manufacturing, significantly impacting electronics.
- Increasing research funding leading to innovative designs and prototypes across labs worldwide.
Fundamental Principles of Plasma Physics
Understanding plasma electricity generation starts with the foundational principles of plasma physics. This area is crucial because it serves as the bedrock for the various techniques and applications that will be discussed later in the article. Without a firm grasp on these principles, one cannot appreciate the complexities and innovative potential of plasma-based energy systems.
Nature of Plasma
Plasma, often referred to as the fourth state of matter, is distinct from solids, liquids, and gases. At its core, plasma consists of a collection of charged particles, primarily ions and electrons. This charged state emerges when enough energy is supplied to a neutral gas, causing atoms to lose or gain electrons. The presence of free-moving charged particles is what categorizes this phenomenon as plasma.
In everyday life, plasmas are not as distant as one might think. Think of neon lights or the flames during a bonfire; each reflects plasma in action. Such instances illustrate how prevalent plasma is, even in mundane settings.
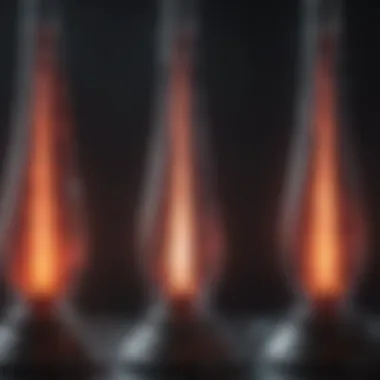
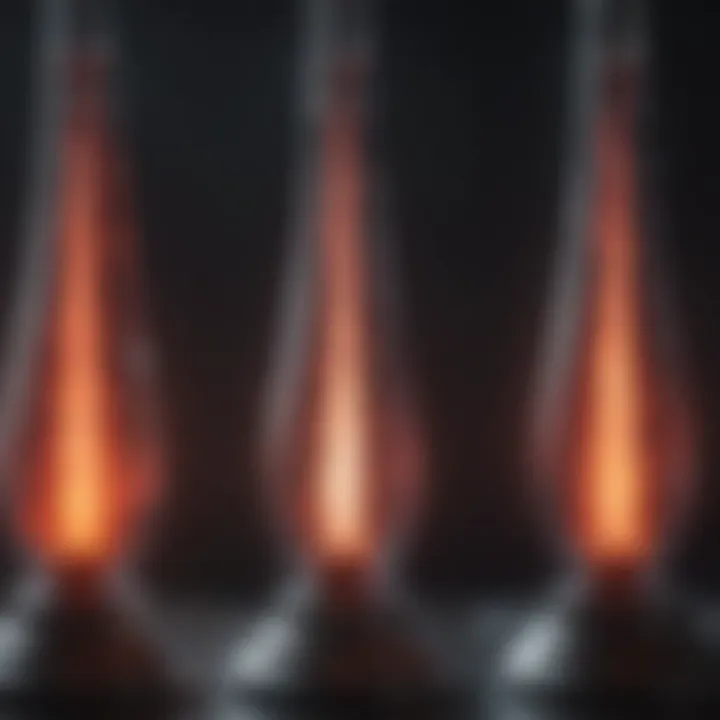
But why does the nature of plasma matter? For one, it can conduct electricity and respond to magnetic fields, opening doors to a myriad of applications stretches far beyond mere lighting.
Ionization Processes
Ionization is the process by which neutral atoms or molecules gain or lose electrons, resulting in charged particles. There are several methods through which ionization can occur, and each one plays a vital role in plasma generation:
- Thermal Ionization: Involves raising the temperature to the point where atoms begin to lose electrons. This can happen in celestial bodies like stars, where their hot interiors produce vast amounts of plasma.
- Photoionization: Occurs when light energy knocks out electrons from atoms. The sun itself is a prime example of this, with solar radiation creating plasma in its atmosphere.
- Collisional Ionization: Takes place when a high-energy particle strikes a neutral atom, causing it to ionize. This is particularly important in various experimental setups aimed at plasma generation.
The ways plasma can be generated via these ionization processes highlight the flexibility and versatility of plasma through different environmental and energetic scenarios.
Characteristics of Plasma
Plasma exhibits unique characteristics that differentiate it from other states of matter. Among these attributes, there are four key traits:
- Electrical Conductivity: Since plasma is composed of charged particles, it can conduct electricity efficiently. This feature is paramount for energy applications where electricity is a focus.
- Response to Magnetic Fields: Due to its charged nature, plasma can be influenced and manipulated by magnetic fields, making it viable for use in technologies like fusion reactors.
- High Temperature: Plasma can reach incredibly high temperatures, surpassing that of gases and providing the energy necessary for fusion reactions, a crucial aspect of plasma-based energy systems.
- Collective Behavior: Plasmas do not behave like individual particles; they act collectively, exhibiting wave-like behavior and phenomena such as plasma oscillations.
Understanding these characteristics is not just academic; it sets the stage for applying plasma in various energy-generating contexts. Knowing how plasma behaves allows engineers and scientists to devise innovative techniques for harnessing its power.
"Plasma, when understood properly, can serve as an unparalleled resource in our quest to redefine energy generation."
In summary, foundational principles of plasma physics are essential for comprehending the mechanisms behind plasma electricity generation. The nature of plasma, the processes that create it, and its distinct characteristics form a comprehensive basis for further exploration of methods and applications in plasma energy.
Methods of Plasma Generation
Methods of plasma generation form the backbone of this study, providing insight into how plasma can be created and utilized for energy production. Each method has its own unique characteristics, advantages, and considerations that significantly impact their application in various fields, especially in energy technology. As the demand for clean and efficient energy sources continues to rise, understanding these methods becomes essential for academics and researchers alike.
Electrical Discharge Techniques
Electrical discharge techniques have emerged as pivotal in the harnessing of plasma electricity generation. These methods typically involve applying a high voltage to a gas, leading to the ionization of the particles within it. An example is the use of a spark discharge, which produces a bright flash of plasma when a high voltage breaks down the air surrounding the electrodes, allowing current to flow.
The method is versatile; it can be adjusted based on the pressure and chemistry of the gas used, facilitating a range of applications from lighting to advanced energy production tools. One key benefit is the relative simplicity and low cost when compared to other generation methods. However, it’s not without its drawbacks. For instance, maintaining stable arcs can be challenging, and the energy efficiency may vary widely based on operational conditions.
- Benefits:
- Considerations:
- Cost-effective
- Relative ease of setup
- Versatile applications
- Stability of the discharge
- Energy efficiency variations
"Electrical discharge techniques have shown promise in both research and practical applications within plasma physics, providing essential groundwork for evolving energy systems."
Laser-Induced Plasma
Moving to laser-induced plasma methods, this approach leverages the intensity of laser beams to ionize gases and create plasma. This technique stands out for its precision, allowing researchers to focus energy on specific targets with remarkable accuracy. When a high-energy laser pulses on a gas, it ionizes the molecules instantly, generating plasma.
The potential applications are numerous. They span from fundamental research in astrophysics to practical applications like material processing, where the emitted plasma can assist in refining materials or even cutting through tough surfaces.
However, this method typically comes with higher operational costs and requires advanced technology for optimal results. The scalability of this approach also presents challenges, especially in larger applications where uniform plasma generation is necessary.
- Strengths:
- Limitations:
- High precision and control
- Suitable for various applications
- High cost
- More complex equipment needed
Microwave Plasma Generation
Finally, we have microwave plasma generation, a technique that utilizes microwave radiation to ionize gases. In this method, microwave radiation excites the gas particles, leading to a rise in temperature and subsequent ionization. This method is particularly noted for producing plasmas at lower pressures, separating it from the previous techniques.
Microwave plasmas can achieve consistent and uniform plasma generation, which is crucial for applications in material sciences and industrial processes. They are frequently employed in processes such as semiconductor manufacturing and plasma-enhanced chemical vapor deposition.
One of the primary draws of microwave plasma is its energy efficiency, achieving a significant yield from the input energy. However, it requires precise control mechanisms and can be complex in terms of equipment and operation.
- Advantages:
- Caveats:
- High energy efficiency
- Consistency in plasma generation
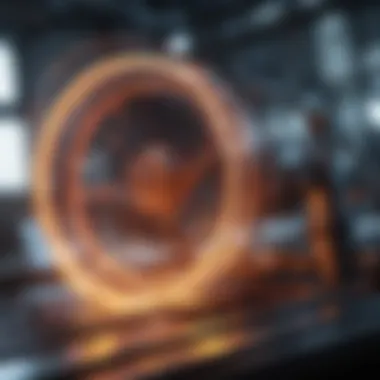
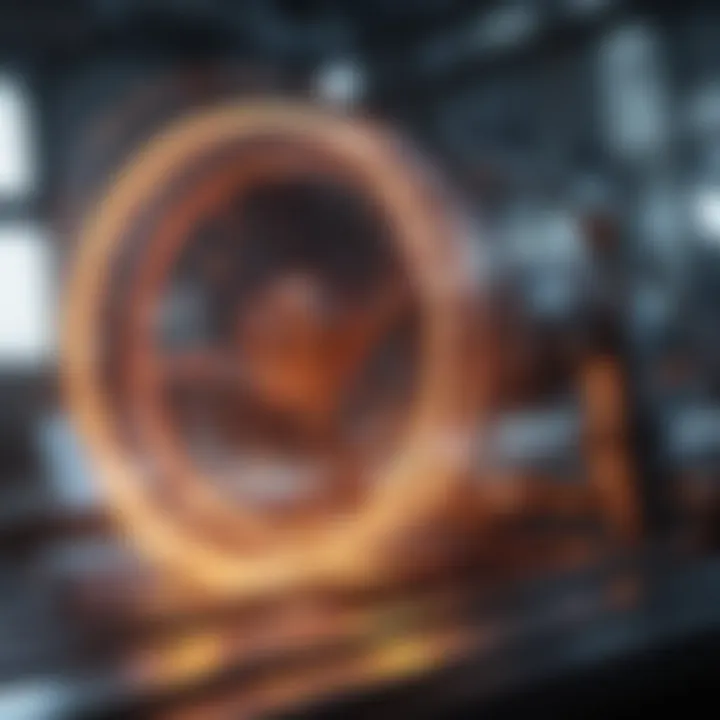
- Equipment complexity
- Control requirements for optimal operation
Application of Plasma in Energy Production
The application of plasma in energy production stands as a lighthouse in the stormy waters of traditional and emerging energy paradigms. This section seeks to dissect the multifaceted roles plasma plays in energy generation, underscoring its potential and the intricacies involved in harnessing its power for practical use. The exploration not only illuminates the benefits and possibilities of these applications but also sheds light on the challenges and considerations that must be navigated.
Nuclear Fusion Research
Nuclear fusion represents one of the most promising avenues for future energy production, closely tied to advances in plasma physics. At its core, fusion is the process that powers the sun, where lighter atomic nuclei join to form heavier ones, releasing a significant amount of energy in the process. This is achieved by creating conditions of extremely high temperature and pressure, often involving plasma.
The importance of nuclear fusion research lies in its potential to provide a nearly inexhaustible source of energy with minimal environmental impact. Here are key specific elements of its significance:
- Sustainable Energy Source: Fusion could meet the world’s energy needs without the pollution and waste associated with fossil fuels.
- Abundant Fuel Supply: Resources such as deuterium, extracted from seawater, and lithium, found in natural ores, offer an ample supply.
However, challenges loom large. The technological limitations, like maintaining a stable plasma state long enough for fusion reactions to occur, require ongoing research and development. The International Thermonuclear Experimental Reactor (ITER) in France is just one initiative aiming to realize this monumental energy shift.
Plasma-Assisted Combustion
Plasma-assisted combustion represents a fusion of traditional combustion practices with plasma technology, striving to boost efficiency and reduce emissions. In simpler terms, integrating plasma into conventional combustion processes can lead to more complete fuel combustion, helping industries to cut back on harmful emissions.
The features of this application include:
- Enhancements in Efficiency: Plasma can ignite fuel mixtures at lower temperatures, allowing for reduced fuel consumption.
- Reduction of Pollutants: The ionized particles in plasma can transform harmful emissions into non-toxic outputs, leading to cleaner combustion cycles.
Consider an example in the automotive industry where plasma is harnessed to improve engine performance; by employing plasma technology, manufacturers can achieve better fuel efficiency and lower emissions. This not only aligns with regulatory pressures for cleaner air but also meets growing consumer demand for environmentally friendly vehicles.
Waste Treatment Technologies
With rising concerns over waste management, plasma technology offers innovative solutions for destroying hazardous waste and biomass. Plasma gasification, in simple words, refers to breaking down organic material at extremely high temperatures, turning waste into syngas, a cleaner and manageable fuel source.
Key advantages of plasma waste treatment technologies include:
- Effective Waste Reduction: Plasma can convert a wide array of waste types, minimizing landfill use.
- Energy Generation: The syngas produced can be used to generate electricity or as a fuel for synthetic fuels, closing the loop on waste disposal.
Studies show that plasma processing leads to a dramatic decrease in pollutants, making this method a potential game-changer for environmental sustainability. By harnessing this technology, cities can take significant strides towards cleaner waste management practices.
"The realm of plasma-based technologies in energy production isn't just about overcoming current challenges; it's about redefining our entire approach to energy sustainability for future generations."
Case Studies on Plasma Energy Systems
The realm of plasma electricity generation is not just theoretical; it’s backed by tangible examples that offer insight into its potential applications and the challenges faced. Case studies serve a vital role in demonstrating how plasma technology is being implemented in real-world scenarios. By analyzing these case studies, we can uncover the strengths and limitations of various plasma systems and understand their place in the future of energy generation.
International Fusion Reactors
Fusion energy, often cited as the holy grail of sustainable energy sources, harnesses the power of plasma. Several international projects are actively pursuing this dream. One of the leading endeavors is ITER (International Thermonuclear Experimental Reactor) based in France. Here, scientists aim to create a self-sustaining fusion reaction by using powerful magnetic fields to confine high-temperature plasma. The ultimate goal is to produce more energy than consumed, a significant leap for mankind's energy approaches.
The scale of ITER is a testament to collaborative international efforts. Countries worldwide are contributing technical expertise, funding, and research resources. However, the complexity of fusion reactors raises questions about material durability. Engineering materials that can withstand extreme conditions of temperature and radiation is an ongoing challenge, requiring continuous innovation.
The success of ITER could pave the way for future commercial fusion reactors. If successful, they would produce massive amounts of energy without contributing to climate change.
Successful Plasma Projects
Even on a smaller scale, there are projects that highlight the efficacy of plasma electricity generation. One notable example is the use of plasma technology in waste-to-energy conversions. By employing plasma arc gasification, waste materials are converted into syngas — a mixture of hydrogen and carbon monoxide that can be used for electricity generation or as a chemical feedstock.
- Plasma Enhanced Melter: This project has been successful in treating hazardous waste. The high temperatures produced from the plasma effectively degrade organic materials. The byproducts are minimal, and the process is efficient.
- Plasma Gasification Plants: Facilities in Canada and the United States have shown how plasma can address the waste crisis by converting landfill waste into valuable energy resources. This dual approach of waste management and energy production is crucial in addressing environmental concerns while utilizing innovative technology.
These successful plasma projects not only validate the technology but also offer a look into the potential economic benefits. The efficiency of plasma systems can lead to operational cost reductions in energy production, providing a significant advantage over traditional methods.
In summary, case studies provide a compelling narrative of plasma energy systems in action. While they showcase the immense potential and collaborative efforts in international projects like ITER, they also highlight the successful implementations of plasma technology in waste management. This exploration into real world applications further underscores the exciting prospects of plasma energy systems.
Challenges in Plasma Electricity Generation
The journey into plasma electricity generation is fraught with obstacles that must be navigated with precision and foresight. Understanding the challenges is imperative, as these issues not only shape current research trajectories but also the long-term viability of plasma as a significant energy source. Notably, the realm of plasma technology is at the intersection of numerous scientific disciplines, thus the challenges presented do not solely stem from one dimension but encompass a broad spectrum including technological, economic, and even societal considerations.
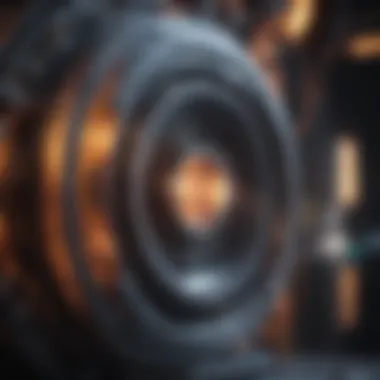
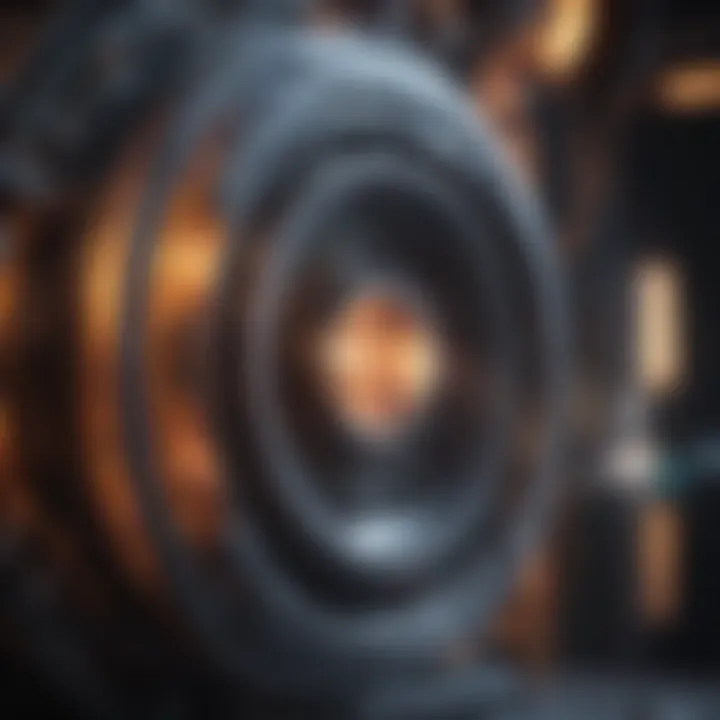
Technology Limitations
At the heart of plasma generation technology lies a multitude of limitations. Chief among these is the issue of stability. Plasma, that elusive fourth state of matter, tends to be tumultuous—finding a method to contain it effectively remains a complex riddle. Magnetic confinement, for instance, utilizes powerful magnetic fields to trap plasma. Yet, imperfections in the magnetic field can lead to instability, resulting in loss of confinement and ultimately disrupting the energy yield.
Moreover, achieving the high temperatures required for efficient plasma generation, particularly in the context of nuclear fusion, demands enormous energy input. The famous Tokamak reactors, like ITER, grapple with this challenge daily. The extensive infrastructure, massive costs, and development timelines also present hurdles that make scaling these technologies for practical use difficult.
The inefficiency in energy conversion from plasma processes to usable electricity is another frequent stumbling block. While theoretically promising, the reality is that many of the processes are presently too inefficient. This inefficiency raises important questions regarding not just technological effectiveness but also where plasma generation can fit into an energy portfolio that includes more established systems.
In summary, while innovation in plasma technology continues apace, its limitations present a complex web of technological hurdles that researchers must overcome to harness plasma effectively.
Economic Viability
The economic viability of plasma electricity generation is anything but straightforward. Investment in this field tends to be hefty, requiring significant funding for both research and development as well as for infrastructure. Potential investors often find themselves on the fence, as the lengthy timelines for return on investment create caution in a world looking for quick solutions to energy demands.
Consider the example of the ITER project in France, which represents a global collaboration aiming to demonstrate the feasibility of fusion energy. The initial budget ballooned far beyond initial estimates, raising eyebrows and concerns over fiscal responsibility. The sheer scale and complexity of such projects necessitate a perpetual balancing act between cutting-edge research and feasible economic models.
Additionally, the competition for funding in the broader energy sector can overshadow plasma generation projects. With renewable sources like wind and solar becoming increasingly cheaper and technologically advanced, plasma investments run the risk of being overshadowed despite their potential.
The societal implications of economic viability cannot be dismissed. Public perception, often driven by the immediate availability and price of energy sources, can diminish interest in plasma systems that may take longer to mature. But as global energy demands grow, compelling narratives that highlight the long-term benefits of investing in plasma technology must be communicated effectively.
In essence, while the potential for plasma electricity generation is expansive, realizing its economic viability is riddled with complexities that must be tackled with a combination of innovative thinking and sound financial strategy.
"The challenges in plasma electricity generation compel us to rethink not just technology, but also our approach to energy as a whole—one where innovation and forward-thinking are indispensable in a rapidly evolving landscape."
Future Prospects of Plasma-Based Energy Systems
The future of plasma-based energy systems holds immense potential, weaving together cutting-edge science and practical applications. As we embark on a journey into this field, it is crucial to appreciate how advancements can affect energy solutions worldwide. This exploration not only seeks to understand the emerging technologies in plasma energy but also assesses their role in a global shift toward sustainable and reliable power sources.
One of the key considerations is the ability of plasma systems to address issues of efficiency and scalability. Traditional energy production methods often fall short in these areas. Conversely, plasma technology focuses on creating high-temperature environments conducive to energy generation. It raises the possibility of producing energy with minimal environmental footprint. In essence, these systems might be the answer to powering our needs without chaining ourselves to fossil fuels and their adverse effects.
Innovations on the Horizon
Innovation in plasma technology is a thrilling aspect of its future. Recent research has birthed new methodologies and devices that enhance the viability of plasma as an energy source. For example, researchers are investigating advanced confinement techniques utilizing magnetic fields. These methods promise greater stability and longer sustainment of plasma, crucial for processes like nuclear fusion.
Here are a few innovations currently on the horizon:
- Small Modular Reactors (SMRs): Leveraging plasma technology, SMRs could deliver cleaner energy to remote areas or microgrids that struggle with traditional methods.
- Improved Electrode Designs: Modern electrodes can significantly impact the efficiency with which plasma is generated and utilized. This includes materials that withstand extreme temperatures, helping to open new pathways in energy creation.
- Hybrid Systems: Integrating plasma generation with existing technologies can create powerful synergies. For instance, combining plasma generation with solar energy can increase overall system efficiency, providing a pathway to more sustainable energy generation practices.
Researchers are also scrutinizing high-energy density materials, which, when exposed to plasma, may lead to unexpected advancements in energy output.
"The shift toward plasma energy is not just a technical feat; it represents a philosophical change in how we view energy production."
Integration with Renewable Energy Sources
Looking further ahead, integrating plasma-based systems with renewable energy holds promise not just for diversification but also for improving grid stability. By blending the volatile nature of solar and wind generation with the robust energy production capabilities of plasma, we can create a reliable energy ecosystem.
Key points to consider regarding this integration include:
- Complementarity: Plasma systems can serve as a stabilizing force, providing power when solar and wind resources fluctuate. This will enhance the reliability of renewable sources, making them more attractive for widespread adoption.
- Decentralization of Energy Production: Plasma systems can be installed in localized setups, reducing transmission losses typically associated with long-distance energy transport. This decentralization approach supports a more resilient power grid.
- Long-term Storage Solutions: One significant challenge in the renewable space is energy storage. Plasma systems offer unique capabilities in energy storage, possibly leading to breakthroughs in how we store excess energy generated during peak production times.
In summary, the future of plasma-based energy systems is framed by innovation and collaboration with renewable technologies. By embracing these advancements, we can foster a sustainable energy landscape capable of meeting the demands of our growing population—and hopefully do so responsibly, with the planet's health front and center.
End and Implications
The exploration of plasma electricity generation is like peering through a lens into the future of energy technology. This article has detailed the fundamental aspects of plasma, from its basic characteristics to the pioneering methods employed to generate it. Understanding plasma not only enhances our knowledge of energy systems but also illuminates pathways for innovation in practical applications. As societies grapple with the heavy weight of climate change and the aging infrastructures of conventional energy sources, the significance of harnessing plasma as a viable energy system becomes glaringly clear.
Summary of Key Findings
Throughout this discourse, several key findings emerge:
- Versatile Applications: Plasma technology serves various fields, ranging from nuclear fusion processes, which hold the promise of safe and clean energy production, to waste treatment systems that help manage hazardous materials efficiently.
- Cutting-Edge Techniques: Different methods of plasma generation, such as laser-induced plasma and microwave plasma generation, mark significant advancements in how we can harness this state of matter. Each technique offers unique advantages depending on the application.
- Economic Considerations: While the economic viability of plasma-based systems is still under investigation, the potential for integrating these technologies with existing renewable energy sources presents exciting prospects for future energy landscapes.
- Research Trends: The current trajectory towards enhanced plasma systems indicates a strong need for interdisciplinary collaboration among scientists and engineers to address the barriers to widespread adoption.
Through these points, it is evident that plasma electricity generation not only holds tremendous promise but is also an intertwined web of challenges and opportunities.
Call for Continued Research and Development
As we stand on the cusp of technological evolution, the call for ongoing research into plasma systems is more pertinent than ever.
- Innovation Beyond Borders: Inviting collaboration across international teams can accelerate breakthroughs. This can bridge the gap between theoretical advancements and their practical implementation.
- Funding and Resources: Governments and private sector entities need to prioritize funding initiatives that support plasma research. With adequate resources, scientists can pursue exploratory projects that, currently, may seem overly ambitious or speculative.
- Education and Training: It's crucial to cultivate a workforce skilled in plasma technologies. Educational programs should incorporate plasma principles so that the next generation of researchers and engineers is well-prepared to tackle the complexities of this field.
In summary, the future of plasma electricity generation hinges on sustained curiosity and relentless inquiry. Only by fostering an environment that encourages continued research and development can we hope to unlock the full potential of this fascinating energy technology.