NAD's Role in DNA Repair Mechanisms Explained
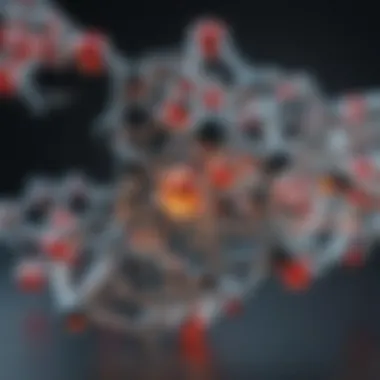
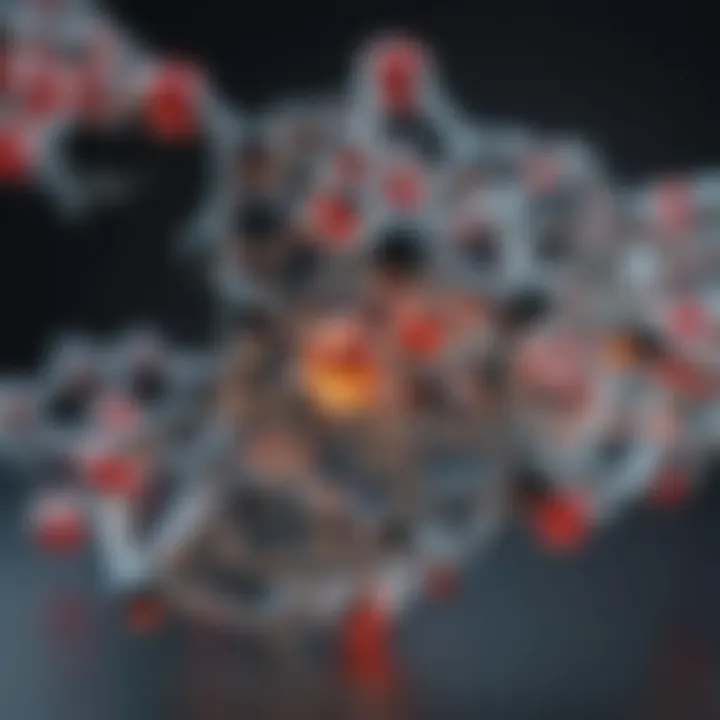
Intro
Nicotinamide adenine dinucleotide (NAD) plays a critical role in various biological processes including cellular metabolism and DNA repair mechanisms. As a coenzyme found in all living cells, NAD is integral to the functioning of many enzymes, particularly those involved in metabolic pathways. The relationship between NAD and DNA repair is particularly complex and significant. This article aims to explore this relationship in detail, analyzing how NAD influences DNA repair processes and the broader implications for cellular health and disease.
Understanding the biochemical mechanisms through which NAD supports DNA repair can shed light on its potential therapeutic applications. This understanding is increasingly relevant as aging populations face a growing burden of age-related diseases and cancer, both of which are often linked to DNA damage and repair deficiencies.
Research Overview
Summary of Key Findings
Recent research highlights several key roles of NAD in DNA repair processes. NAD serves as a substrate for enzymes such as poly(ADP-ribose) polymerases (PARPs), which are essential for repairing single-strand breaks in DNA. Furthermore, NAD is involved in sirtuins, a family of proteins that deacetylate proteins associated with DNA repair and stress responses. The findings from various studies indicate that a sufficient level of NAD is crucial for maintaining the efficacy of DNA repair mechanisms.
Significance of Research
Exploring NAD's role in DNA repair is significant because it opens avenues for developing therapeutic strategies. Enhancing NAD levels or mimicking its functions could be a strategy to improve DNA repair capabilities in aging cells. This has particular relevance for cancer treatments, where DNA repair processes can dictate the effectiveness of therapies. Understanding these connections may lead to novel interventions aimed at bolstering cellular integrity and functionality.
Methodology
Research Design
In conducting this exploration, a systematic review of current literature was undertaken. Studies investigating NAD's role in DNA repair mechanisms were analyzed, focusing on biochemical pathways and empirical findings associated with cellular health.
Data Collection Methods
Data were collected primarily from peer-reviewed journal articles, biochemical studies, and clinical research reports. Emphasis was placed on gathering quantitative data related to NAD concentrations, DNA damage repair rates, and outcomes from interventions designed to modify NAD levels. This comprehensive approach facilitates a nuanced understanding of how NAD influences DNA repair and cellular homeostasis.
"The importance of NAD in DNA repair can not be underestimated. Its role as a cofactor in essential repair enzymes marks a crucial point of interest for both researchers and clinicians." - Research Overview
By synthesizing these findings, the next sections will delve into specific mechanisms of DNA repair influenced by NAD, highlighting pathways, enzyme roles, and implications for health.
Preface to NAD
Nicotinamide adenine dinucleotide, or NAD, is a crucial coenzyme present in all living cells. Understanding NAD is essential for comprehending its role in cellular processes and its significance in DNA repair mechanisms. The importance of NAD extends beyond simple metabolism. It is deeply intertwined with maintaining genome integrity, which underlies various cellular functions, including the regulation of cellular health, energy metabolism, and aging.
A growing body of research indicates that NAD levels can impact how cells respond to DNA damage, a fundamental aspect of cellular biology. This connection becomes particularly relevant in the context of diseases associated with aging and cancer, where DNA repair mechanisms often become impaired. Thus, exploring the biochemical and functional aspects of NAD will provide a comprehensive framework for understanding its pivotal role in preserving cellular and genomic health.
Biochemical Structure of NAD
NAD consists of two nucleotides joined by their phosphate groups. One nucleotide contains an adenine base, while the other has a nicotinamide base. This simple yet effective structure allows NAD to participate in redox reactions as an electron carrier, playing a vital role in oxidative metabolism. Additionally, NAD exists in two forms: NAD+ (oxidized) and NADH (reduced). This interconversion is central to various metabolic pathways, particularly those that generate ATP from nutrients.
NAD’s structure allows it to participate in numerous enzymatic reactions involved in energy production and repair mechanisms. The presence of the nicotinamide moiety enables NAD to act as a substrate for important enzymes involved in DNA repair pathways.
Synthesis and Metabolism of NAD
NAD synthesis occurs primarily through three pathways: the de novo synthesis pathway, the salvage pathway, and the NAD+ recycling pathway. The de novo pathway starts with the amino acid tryptophan, while the salvage pathway recycles nicotinamide derived from NAD degradation. This recycling is crucial because it helps maintain adequate NAD+ levels under various metabolic conditions.
The metabolism of NAD is tightly regulated and involves several enzymes, including nicotinamide nucleotide adenylyltransferase and nicotinamide adenine dinucleotide glycohydrolases. These enzymes are essential for balancing cellular NAD levels, ensuring that NAD is available for both energy metabolism and DNA repair processes. Disruptions in NAD metabolism can lead to decreased availability, which impacts cellular health and the ability to repair DNA damage.
NAD's Role in Cellular Processes
NAD plays a multifaceted role in cellular biology. Beyond its function as a coenzyme in energy production, NAD is also involved in signaling pathways and gene expression regulation through its role in NAD+-dependent enzymes, such as sirtuins and PARPs. Sirtuins, for example, use NAD as a substrate to deacetylate histones, thus playing a key role in gene regulation and cellular health.
Moreover, NAD is crucial in stress response and aging processes. It influences the cellular response to oxidative stress and helps maintain metabolic functions under adverse conditions. Adequate NAD levels promote longevity and reduce the risk of age-related diseases by facilitating efficient DNA repair pathways and cellular repair mechanisms.
In summary, understanding NAD in its various aspects—from structure to metabolic pathways—sets the groundwork for exploring its instrumental role in DNA repair and cellular processes.
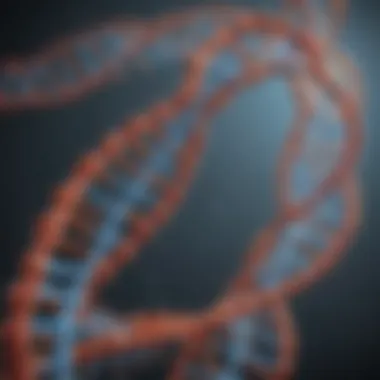
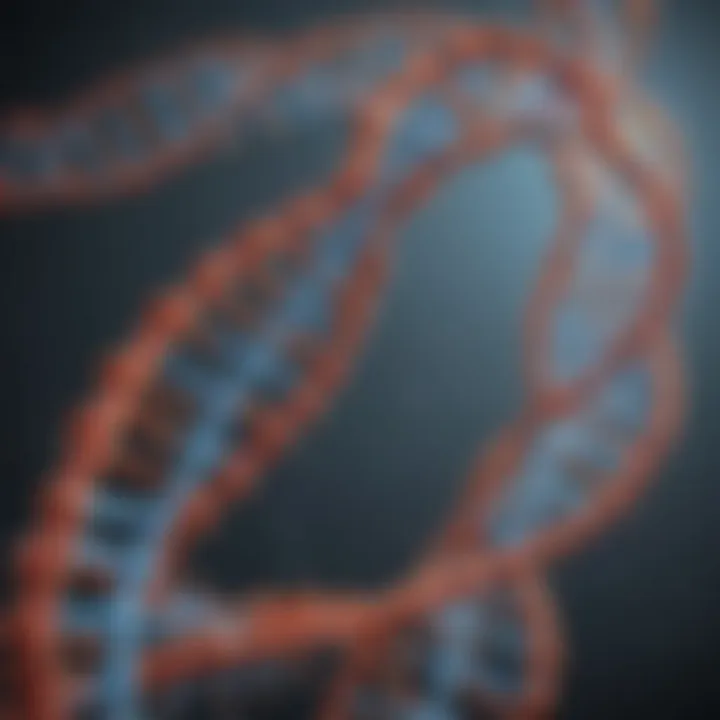
DNA Structure and Function
Understanding the structure and function of DNA is crucial in the context of NAD's role in DNA repair mechanisms. DNA is the genetic blueprint for all living organisms, carrying the information necessary for growth, development, and reproduction. The stability and integrity of DNA are vital for maintaining cellular functions. Consequently, any damage to DNA can lead to severe consequences, including mutations, cancer, and aging. DNA's relationship with NAD becomes significant when considering how cells respond to such damage. The efficiency of DNA repair processes can directly influence cellular health, making it essential to explore DNA's structural and functional aspects.
Overview of DNA Structure
DNA, or deoxyribonucleic acid, has a double helix structure, composed of two intertwined strands that resemble a twisted ladder. This iconic configuration is due to the arrangement of nitrogenous bases (adenine, thymine, cytosine, and guanine) that pair specifically: adenine pairs with thymine and cytosine pairs with guanine. The backbone of the DNA strand consists of alternating sugar and phosphate groups. This arrangement forms the structural foundation, ensuring the stability and accessibility of the genetic code.
The double helix is stabilized by hydrogen bonds between the base pairs and by hydrophobic interactions among the stacked bases. These features allow DNA to pack tightly within cellular confines while remaining accessible for replication and transcription processes. The specific sequences of these bases encode the information required for protein synthesis, cell function, and overall biological processes. Thus, understanding DNA’s architecture provides insights into how genetic information is stored and utilized within cells.
Functions of DNA in Cellular Biology
DNA serves several essential functions within cellular biology. Primarily, it acts as the information repository for living organisms, storing instructions for developing new cells and organisms. Here are several key functions:
- Replication: Before a cell divides, DNA must replicate to ensure that each daughter cell receives an exact copy of the genetic information.
- Transcription: DNA serves as a template for synthesizing RNA, which plays a crucial role in protein production. This process is vital for cellular structure and function.
- Regulation: Specific sequences within DNA govern when genes are expressed, allowing cells to respond dynamically to their environment.
- Repair: DNA possesses intrinsic mechanisms for detecting and correcting errors. This is where NAD comes into play, as it influences various DNA repair enzymes crucial for maintaining genomic integrity.
In summary, the structure of DNA not only dictates its function but also highlights the vital role of NAD in repair mechanisms. When DNA is damaged, understanding its structure and biology can inform approaches to therapeutic issues related to aging and disease.
Types of DNA Damage
Understanding the types of DNA damage is vital to appreciating how NAD influences DNA repair mechanisms. DNA, the blueprint of life, is constantly exposed to various stressors that can compromise its integrity. Types of DNA damage can be broadly categorized into physical, chemical, and biological impacts. Each category presents specific challenges and consequences for cellular health.
Physical and Chemical Damage
Physical and chemical damage to DNA arises from external and internal sources. This can include environmental factors, such as UV radiation and chemicals in food or air. UV radiation can cause pyrimidine dimers, where two adjacent thymine bases bond abnormally, distorting the DNA structure. On the other hand, chemical agents can result in a myriad of modifications, including base substitutions or strand breaks.
- Ionizing Radiation: This type of radiation can create free radicals, leading to oxidative damage. Free radicals can react with DNA, causing strand breaks or base modifications.
- Chemicals: Substances like benzene or formaldehyde can interact with DNA, resulting in alkylation changes. Unchecked, these alterations can lead to mutations, triggering diseases like cancer.
- Oxidative Stress: A byproduct of normal metabolism, oxidative stress can also lead to the production of reactive oxygen species (ROS). ROS are notorious for causing substantial DNA damage, ultimately leading to cellular dysfunction.
The repair of physical and chemical damage is crucial for cell survival and function. Just like a current of water wears down rock over time, continuous damage requires constant attention from cellular repair pathways. NAD plays a pivotal role in modulating the efficiency of these pathways, particularly those involved in detecting and fixing such injuries.
Biological Consequences of DNA Damage
The consequences of DNA damage extend far beyond immediate cell survival. When damage occurs, the biological consequences can affect cellular behavior, leading to various outcomes.
- Cell Cycle Arrest: Damage may result in the halting of the cell cycle, allowing the cell to repair itself before proceeding to divide.
- Apoptosis: In cases of irreparable damage, cells can initiate a programmed death process to prevent the propagation of mutations, which is a protective mechanism.
- Mutagenesis: If repair mechanisms fail or are insufficient, mutations can accumulate over generations. This accumulation is a significant factor in aging and the development of diseases.
"The cumulative impact of such damage underscores the significance of effective repair systems in preserving genomic integrity, with NAD playing a critical supportive role."
Understanding these types and consequences of DNA damage not only reveals insights into cellular health but also highlights the importance of NAD within the intricate web of repair mechanisms. Each form of damage necessitates a specific response, and the role of NAD in enhancing enzymatic activity and supporting cellular repair processes cannot be overstated. Identifying and studying these dynamics can pave the way for advanced therapeutic strategies aimed at preserving DNA integrity and cellular function.
DNA Repair Mechanisms
DNA repair mechanisms are essential for maintaining genomic integrity and preventing mutations that can lead to various diseases, including cancer. The importance of these processes lies in their ability to correct damage caused by environmental factors, metabolic byproducts, and even normal cellular activities. DNA is continuously subjected to damage, hence effective repair mechanisms are crucial for cellular health and longevity. Understanding these mechanisms will provide insights into therapeutic strategies that can exploit or enhance repair processes, particularly in the context of aging and disease.
Base Excision Repair
Base excision repair (BER) is a critical pathway that resolves DNA damage resulting from oxidation, deamination, and alkylation. This seemingly simple yet sophisticated process focuses on removing damaged bases from DNA and replacing them with the correct ones. BER is initiated when a DNA glycosylase recognizes and removes the damaged base, creating an abasic site. This is followed by an endonuclease that cuts the strand, allowing DNA polymerase to insert the correct nucleotide. Finally, DNA ligase seals the nick to restore DNA continuity.
Given the high prevalence of base damage in DNA, the efficiency of BER is vital for cellular health. Certain factors can influence the efficiency of this process, especially NAD levels. NAD directly influences the activity of several enzymes involved in DNA repair pathways, highlighting its role in maintaining correct base pairings and overall genomic stability.
Nucleotide Excision Repair
Nucleotide excision repair (NER) is another essential mechanism that addresses bulky DNA adducts and helix-distorting lesions caused by UV radiation and certain chemical exposures. NER involves the recognition of the damage, unwinding of the DNA, and excising a short single strand that contains the lesion. This process requires multiple proteins to function correctly, namely, damage recognition proteins, helicase, and exonuclease.
Once the damaged section is removed, DNA polymerase fills in the gap with new nucleotides, and DNA ligase completes the repair. The efficiency of NER can be critically affected by NAD levels, as NAD-dependent enzymes play pivotal roles in the signaling and execution of the repair process. Understanding how NAD influences NER can provide insights into how to enhance repair efficiency in aging cells or those under stress.
Double-Strand Break Repair
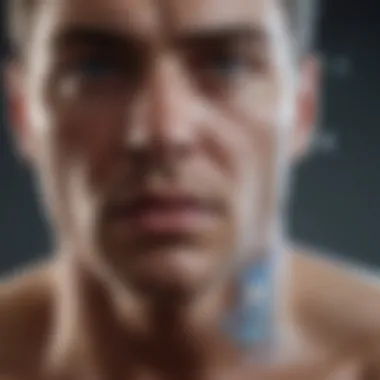
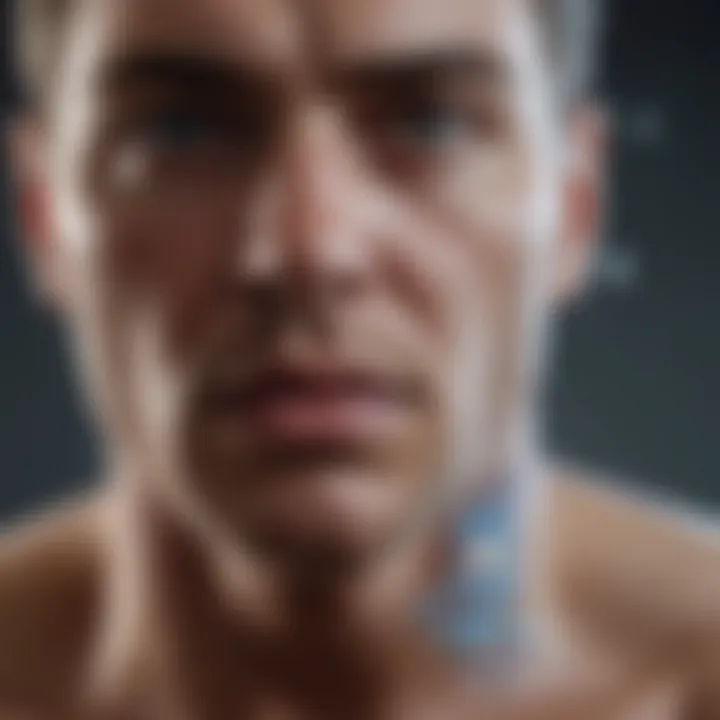
Double-strand breaks (DSBs) represent one of the most severe forms of DNA damage, potentially leading to cell death or cancer if not repaired swiftly and accurately. Two primary repair pathways address DSBs: homologous recombination (HR) and non-homologous end joining (NHEJ). HR is a precise mechanism that uses a homologous template for accurate repair, while NHEJ joins broken ends directly, which can sometimes be error-prone.
The choice between these repair pathways is influenced by the cell cycle stage, and it depends on the presence of factors such as NAD. Recent studies show that NAD levels can affect the choice of repair mechanism; high NAD may promote HR, which is usually more accurate. This relationship underscores the importance of NAD in maintaining genomic stability by facilitating efficient repair of DSBs. Strategies that enhance NAD availability may improve the fidelity of DSB repair, affecting cancer treatment responses and cellular aging processes.
Role of NAD in DNA Repair
The role of nicotinamide adenine dinucleotide (NAD) in DNA repair is critical for maintaining cellular integrity and function. NAD is a coenzyme present in all living cells and is essential for various metabolic processes. In the context of DNA repair, it serves more than just a supporting role. Its influence extends to the regulation of numerous repair enzymes, making it indispensable for the proper repair of damaged DNA.
NAD plays a vital role in two primary ways:
- Influencing DNA repair pathways: NAD is a substrate for several enzymes instrumental in the DNA repair processes, including poly(ADP-ribose) polymerases (PARPs). These enzymes are activated upon detecting DNA damage, thus underscoring the significance of NAD in orchestrating prompt cellular responses to repair mechanisms.
- Maintaining cellular energy: Through its role in cellular metabolism, NAD contributes to the maintenance of energy levels necessary for DNA repair. A well-functioning repair mechanism requires adequate energy supply to mobilize repair proteins and perform the repair processes efficiently.
Overall, NAD is an essential factor in the context of DNA repair. Its regulatory capacity on key enzymes ultimately facilitates the recovery of genetic material from damage, which is vital for cellular health and longevity.
NAD's Influence on Repair Enzymes
NAD has a profound influence on the functionality of various repair enzymes involved in different DNA repair pathways. For example, PARP enzymes utilize NAD as a substrate to synthesize poly(ADP-ribose), a vital molecule that helps signal and recruit other necessary repair proteins to sites of DNA breaks.
The activation of PARPs occurs swiftly upon the detection of DNA damage. This process initiates a cascade of cellular responses that contribute to the repair of the DNA strands. In addition, higher NAD levels enhance these activities, promoting efficient and timely repair of the DNA, which is crucial to prevent genomic instability.
Moreover, the interaction between NAD and DNA repair enzymes does not only involve PARPs. Other repair mechanisms, such as base excision repair and nucleotide excision repair, also benefit from NAD's presence. Its role acts more like a regulatory agent that ensures enzymes operate effectively during crucial repair processes. Without sufficient NAD available, the activation and subsequent functionality of these enzymes may be impaired, risking cellular health and functionality.
NAD Depletion and Its Effects on Repair Mechanisms
Depleting NAD levels within cells can have significant consequences for DNA repair mechanisms. When NAD is reduced, the enzymatic activity of key DNA repair proteins diminishes, leading to a decreased ability to repair DNA damage effectively.
NAD depletion can occur due to several factors, including aging, metabolic disorders, or a high-energy demand in tissues. The consequences of low NAD levels may manifest in multiple ways:
- Increased genomic instability: Cells may accumulate DNA damage due to ineffective repair mechanisms, resulting in mutations and chromosomal aberrations.
- Enhanced susceptibility to cellular stress: Decreased NAD levels can lead to stress responses and eventual cell death due to the inability of cells to manage DNA repair and maintain cellular functions.
- Worsening aging processes and age-related diseases: Sustained low levels of NAD have been correlated with biological aging and the onset of age-related diseases, as cells become less resilient to damage.
NAD and Cellular Senescence
The study of NAD, or nicotinamide adenine dinucleotide, in relation to cellular senescence presents intriguing insights into the aging process. Senescence refers to the state where cells cease to divide but remain metabolically active. This state can have significant implications for tissue function and overall health. Understanding how NAD levels influence this process is vital, as it may provide pathways to mitigate age-related decline and enhance cellular repair mechanisms.
Connection Between NAD Levels and Aging
NAD levels decline with age, contributing to various aging-related disorders. This depletion affects many cellular processes, especially those involving energy metabolism and DNA repair. When cells age, the reduction in NAD makes it harder for them to maintain homeostasis and respond to damage.
Studies show that replenishing NAD levels can help restore cellular functions, potentially improving lifespan and healthspan. For instance, the administration of NAD precursors like nicotinamide riboside or nicotinamide mononucleotide has demonstrated promise in preclinical trials. These compounds can elevate NAD levels in tissues, thereby stimulating pathways important for DNA repair and cellular defense.
"Maintaining NAD levels is crucial for preserving the functionality of cells as they age."
NAD's Role in Senescence-related DNA Damage
Cellular senescence is often accompanied by an accumulation of DNA damage. This damage can result from various stressors, including oxidative stress and telomere shortening. NAD plays a critical role in repair pathways that address such damage.
Aging cells with low NAD levels may struggle to activate NAD-dependent enzymes like PARP (poly(ADP-ribose) polymerase), which are essential for the repair of single-strand breaks in DNA. Insufficient NAD not only compromises the efficiency of these repair mechanisms but also contributes to genomic instability, a hallmark of aging.
In summary, understanding the connection between NAD and cellular senescence provides insights into the aging process itself. By exploring this relationship, researchers can develop targeted interventions that may slow the aging process and enhance the body’s ability to repair DNA damage.
Therapeutic Implications
Understanding the therapeutic implications of NAD is crucial for applications in both age-related diseases and cancer treatment. NAD is not just a coenzyme; it serves a multifunctional role that impacts cell metabolism and repair mechanisms. Given its involvement in various enzymatic reactions and pathways, targeting NAD levels could have significant ramifications for enhancing cellular function and repairing DNA. The interplay between NAD and DNA repair mechanisms offers a promising therapeutic avenue to address issues related to genomic stability.
One specific aspect worth noting is the effect of NAD depletion. As cells lose NAD through aging or stress, their ability to promptly repair DNA becomes compromised. This can lead to mutations and eventually result in diseases like cancer. Therefore, maintaining optimal NAD levels may support the body’s intrinsic capacity to manage DNA damage. Researchers are increasingly interested in how modulating NAD can lead to better treatment outcomes, particularly for conditions associated with aging.
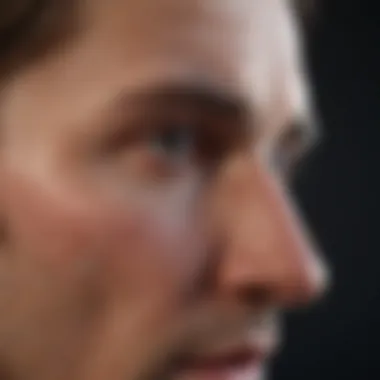
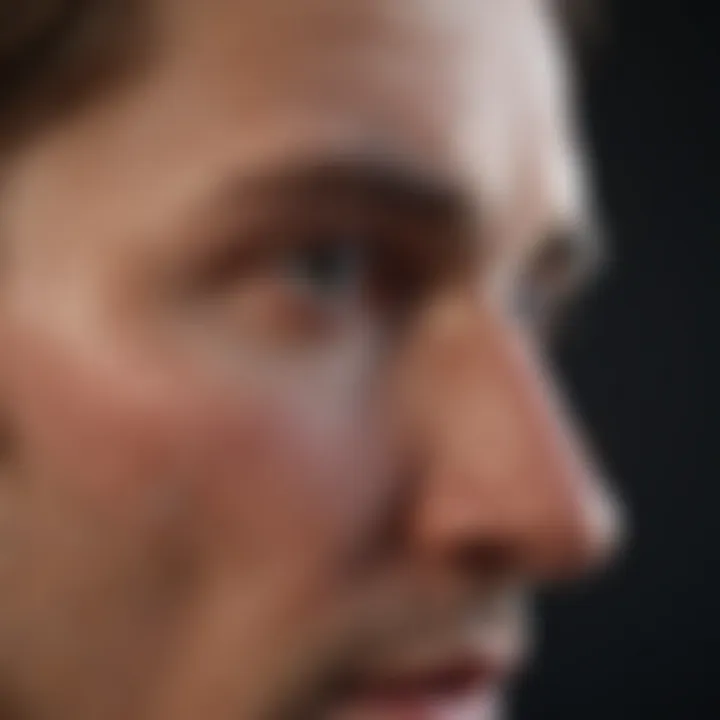
There are several considerations regarding the therapeutic applications of NAD in clinical settings, which include:
- NAD precursors: Supplements like nicotinamide riboside and nicotinamide mononucleotide have gained attention for their potential to replenish NAD levels.
- Synergistic effects: Combining NAD precursors with existing therapies may enhance efficacy and reduce side effects.
- Patient variability: Individual responses to NAD modulation may vary based on genetic makeup and existing health conditions.
To summarize, NAD-boosting interventions could have wide-ranging benefits in treating not only cancer but also conditions tied to cellular aging, modulating the rates of DNA repair and consequently impacting overall cellular health.
NAD Precursors in Treatment
Research into NAD precursors reveals significant potential in therapeutic strategies. Nicotinamide riboside and nicotinamide mononucleotide are the most studied NAD precursors. These compounds can effectively raise NAD levels in human cells, supporting various physiological processes. In clinical settings, these precursors could offer new options for treating diseases characterized by impaired DNA repair and high levels of oxidative stress.
NAD precursors work by:
- Increasing availability of NAD: These compounds enter cells and get converted into NAD, thereby boosting its levels.
- Improving mitochondrial function: Enhanced NAD levels support mitochondrial health, which is critical for ATP production and cellular energy.
- Mitigating age-related decline: Studies suggest that replenishing NAD can potentially slow down age-associated cellular decline, thus having implications for longevity.
In practice, integrating NAD precursors into treatment plans may also promote better responses to other therapies.
NAD and Cancer Therapy
The relationship between NAD and cancer therapy is complex but increasingly recognized as a critical area of study. Cancer cells typically exhibit altered metabolism, and many of them display increased reliance on NAD for survival and proliferation. Understanding this dependence could open avenues for targeted therapies aimed at cancer cells’ unique metabolic vulnerabilities.
NAD’s dual role can be summarized as:
- Promoting cell survival: Cancer cells often exploit NAD to enhance their lifespan and resist apoptosis.
- Facilitating DNA repair: Ironically, while NAD boosts repair mechanisms, this can also lead to resistance against conventional treatments such as chemotherapy and radiation.
Considering these dynamics, therapeutic strategies involving NAD modulation may:
- Inhibit cancer cell proliferation: Lowering NAD might reduce tumor growth, as cancer cells often rely on high levels of NAD.
- Enhance sensitivity to treatment: By targeting NAD-dependent mechanisms, therapies could make cancer cells more susceptible to existing treatments such as chemotherapy.
Future Research Directions
The area of NAD and DNA repair mechanisms presents numerous opportunities for further exploration. This is critical as advancements may lead to a deeper understanding of cellular processes and the implications for therapeutic applications. Researchers are increasingly interested in how NAD not only supports energy metabolism but also plays a significant role in maintaining genomic integrity. Exploring the intricate relationships and mechanisms that are currently unexplored could yield promising pathways for treatment in age-related diseases and cancers.
Unexplored Mechanisms of NAD in DNA Repair
Recent studies have focused primarily on the established roles of NAD in DNA repair, such as its function in regulating enzymes involved in base excision repair or double-strand break repair. However, the mechanisms by which NAD influences other forms of repair and cellular stress responses remain largely uncharted. Understanding how NAD interacts with other cofactors and cellular signaling pathways can open new avenues for research.
Research may need to encompass the following:
- Cross-talk with different metabolic pathways: Investigating how NAD metabolism interacts with nutrient sensing pathways, like mTOR and AMPK, could show how cells adapt to stress.
- Effects of NAD on chromatin remodeling: Analyzing whether NAD levels influence chromatin structure during DNA repair processes might reveal novel regulatory mechanisms.
- The role of NAD in mitochondrial DNA repair: The involvement of NAD in maintaining both nuclear and mitochondrial DNA integrity is under-researched. Understanding this role is crucial since mitochondrial dysfunction is linked to various diseases.
These aspects suggest that NAD has a deeper, multifaceted role in DNA repair processes that warrants more in-depth investigation.
Potential for Interdisciplinary Studies
Interdisciplinary research is crucial for fostering innovative solutions and breakthroughs in understanding NAD and DNA repair mechanisms. Bringing together experts from various fields—such as biochemistry, molecular biology, and medicine—can facilitate a comprehensive approach towards addressing complex biological questions.
Some areas that could benefit from interdisciplinary collaboration include:
- System biology and modeling: Employing computational models to simulate NAD metabolism could lead to significant insights about its impact on DNA repair dynamics.
- Clinical research partnerships: Collaboration between researchers and healthcare providers could facilitate the translation of basic research findings into clinical applications.
- Chemical studies on NAD analogs: Chemistry research on synthetic NAD precursors or analogs may enhance the understanding of NAD’s role in DNA repair and provide new therapeutic options.
Culmination
The conclusion of this article reinforces the nuanced interplay between nicotinamide adenine dinucleotide (NAD) and DNA repair mechanisms, a relationship that warrants careful consideration in the fields of molecular biology and therapeutic development. The discussions throughout the article highlight that NAD is not merely a metabolic cofactor but a pivotal player in the fidelity of cellular DNA repair processes.
Understanding the importance of NAD in DNA repair mechanisms underscores various specific elements. Firstly, its regulatory influence over key enzymes involved in repair pathways emphasizes NAD's role as a potential target for therapeutic interventions. By manipulating NAD levels or its precursors, researchers may conceptualize innovative strategies for treating age-related diseases and minimizing the risks associated with genomic instability.
Moreover, the implications of NAD's functionality extend to cancer biology. Cancer cells often exhibit altered NAD metabolism, leading to impaired DNA repair capabilities. Thus, enhancing NAD availability can reinstate normal repair function, potentially curtailing tumorigenesis.
Key benefits arise from acknowledging the role of NAD in DNA repair. Researchers and clinicians can improve treatments for conditions linked to impaired repair mechanisms, such as neurodegenerative diseases and certain cancers. This focus lays the groundwork for interdisciplinary exploration, linking biochemistry and clinical medicine more closely than has previously been pursued.
As we advance in our understanding, it is imperative to consider the broader context in which NAD operates. Future studies can focus on unexplored mechanisms by which NAD influences DNA repair processes and assess how aging affects NAD metabolism. This could drive significant progress toward innovative therapeutic approaches.
In summary, the relationship between NAD and DNA repair mechanisms is a critical discourse that integrates various dimensions of biology. Insights from this article not only contribute to academic knowledge but also guide practical applications in health and medicine, offering a robust framework for future research.