In-Depth Analysis of PCR Testing in Diagnostics
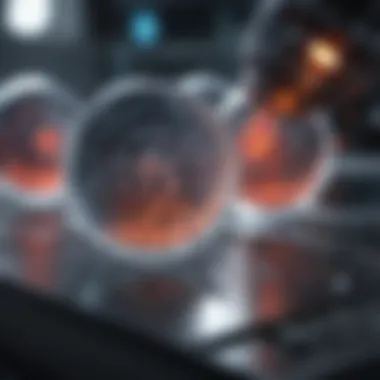
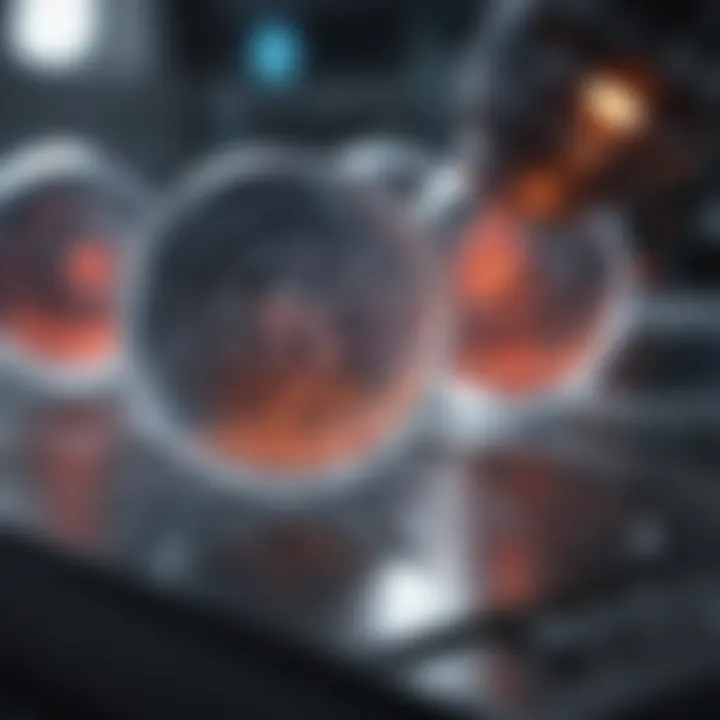
Intro
The Polymerase Chain Reaction (PCR) test has reshaped the landscape of molecular diagnostics, making it a cornerstone in the toolkit of modern medicine. In today’s world, where the urgency for rapid and accurate diagnostic methods is greater than ever, understanding the intricacies of PCR becomes essential. This article dives into the biological foundations, applications, and advancements of PCR, all while elucidating its vital significance in medical diagnostics.
Research Overview
The profound impact of PCR in various fields cannot be overstated. In this section, we will summarize the key findings surrounding the technology and highlight its significance within the realm of health care.
Summary of Key Findings
- Versatility in Pathogen Detection: PCR can identify a variety of pathogens, including bacteria, viruses, and fungi. Its ability to amplify specific DNA sequences allows for the detection of pathogens even in minimal concentrations.
- Rapid Results: One of the chief advantages of PCR is the speed with which results can be obtained. Traditional culturing methods, which may take days or even weeks, can be supplanted with PCR testing that yields results within hours.
- High Sensitivity and Specificity: The test boasts a high degree of both sensitivity and specificity, significantly reducing the possibility of false negatives or positives, thus making it reliable for clinical diagnostics.
- Advancements in Technology: The evolution of PCR methods, including quantitative PCR (qPCR) and digital droplet PCR (ddPCR), has expanded the applications of this technology beyond infectious disease diagnostics to include genetic research and cancer diagnosis.
Significance of Research
The implications of PCR in diagnostics can be extensive:
- Public Health Impact: By enabling swift identification of pathogens, PCR plays a crucial role in controlling outbreaks. It allows health officials to respond promptly, curtailing the spread of infectious diseases.
- Personalized Medicine: Developing targeted therapies based on genetic testing has become more feasible through PCR. By understanding the specific genetic makeup of diseases, healthcare providers can tailor treatments to individual patients, improving outcomes.
"PCR tests have not just changed the way we diagnose diseases, they have revolutionized the approach of personalized medicine."
Methodology
To fully grasp why PCR is a valuable tool in diagnostics, it is important to understand how the methods are structured and conducted.
Research Design
The research design typically involves both laboratory methods and field studies to assess the efficacy of PCR in real-world scenarios. Controlled experiments are carried out to validate its diagnostic capabilities, followed by extensive clinical trials.
Data Collection Methods
Colleced data through PCR examinations may involve:
- Sample Collection: Swabs from nasal passages, blood samples, and tissue biopsies are common.
- Amplification Process: The DNA extracted from the samples undergoes amplification cycles to facilitate analysis.
- Interpretation: Results are carefully interpreted, often with additional confirmation via sequencing or alternative testing methods, ensuring the reliability of outcomes.
With these frameworks, the article will further explore the nuances of PCR technology in upcoming sections, expanding on its applications in clinical settings and discussing ongoing advancements.
Foreword to PCR Testing
PCR testing serves as a cornerstone of modern molecular diagnostics. It allows for the rapid detection of pathogens, facilitating timely clinical interventions. The importance of introducing the topic of PCR testing lies in its ability to transform sample traces of genetic material into quantifiable results. As health threats evolve, understanding the depths and breadths of this technology becomes indispensable.
The Polymerase Chain Reaction, or PCR, is pivotal in numerous fields, ranging from clinical diagnostics to forensic analysis. Utilizing PCR, healthcare professionals can identify an extensive array of pathogens, including bacteria, viruses, and even some fungi and parasites. The implications for public health are profound; early detection can lead to better outcomes.
The Concept of PCR
At its core, PCR is a method that amplifies small segments of DNA, enabling the analysis of genetic material found in various samples. It essentially replicates targeted DNA sequences exponentially, which can then be detected and analyzed. Imagine needing to find a specific needle amongst a haystack; PCR finds and amplifies that needle so it's more visible.
The process typically involves three primary steps: denaturation, annealing, and extension.
- Denaturation: This is where the double-stranded DNA is heated until the strands separate.
- Annealing: Short primers that correspond to the DNA sequence of interest attach to the specific site.
- Extension: An enzyme called Taq polymerase helps to synthesize a new strand of DNA by extending from the primers.
This cyclical process results in exponential amplification of the specific DNA segment.
Historical Development of PCR
The inception of PCR in 1983 by Kary Mullis marked a revolutionary shift in the way scientists approached genetic analysis. Mullis, while working as a biochemist, recognized the potential for amplifying specific DNA sequences efficiently. Since his initial discovery, this technique has evolved significantly.
Over the years, modifications and enhancements have been introduced to improve the efficiency, specificity, and speed at which PCR can be performed. Early challenges, such as primer design and contamination issues, have been mitigated through advanced techniques like real-time PCR.
Today, PCR is not just a laboratory routine but a fundamental tool within diverse areas, such as diagnostic testing, environmental monitoring, and even in our legal systems for forensic evidence. As such, comprehending the historical context of PCR enables researchers and practitioners to appreciate the technological advancements made and the pathway forward for molecular diagnostics.
The Biological Mechanism of PCR
The Biological Mechanism of PCR is a cornerstone of molecular diagnostics, as it unveils how the technique amplifies specific DNA sequences. Understanding this mechanism is vital for grasping the PCR test's efficiency, reliability, and applications in various fields such as medicine, forensic science, and environmental testing. The ability to pinpoint and amplify tiny amounts of DNA has transformed diagnostic practices, enabling researchers and clinicians to detect pathogens at astonishing sensitivity.
DNA Amplification Process
The DNA amplification process is where the magic of PCR truly happens. To put it simply, PCR mimics natural DNA replication but on a much faster scale. During this process, a target segment of DNA is exponentially duplicated, leading to millions, if not billions, of copies.
- Initiation: The first step involves denaturation, where the double-stranded DNA is heated to about 94-98 degrees Celsius. This heat causes the DNA strands to separate, akin to pulling apart a zipper.
- Annealing: After denaturation, the temperature is lowered to around 50-65 degrees Celsius. At this cooler temperature, short segments of nucleotides known as primers attach to the specific regions of the DNA template. Think of primers as hooks, ensuring that the next step proceeds properly.
- Extension: The final step is the extension phase, where the temperature is raised to about 72 degrees Celsius. Here, the enzyme Taq polymerase adds nucleotides to the growing DNA strand based on the template provided. The cycle is repeated multiple times, leading to exponential amplification of the target DNA.
This meticulous orchestration allows PCR to rapidly produce ample amounts of DNA suitable for analysis.
Role of Primers and Enzymes

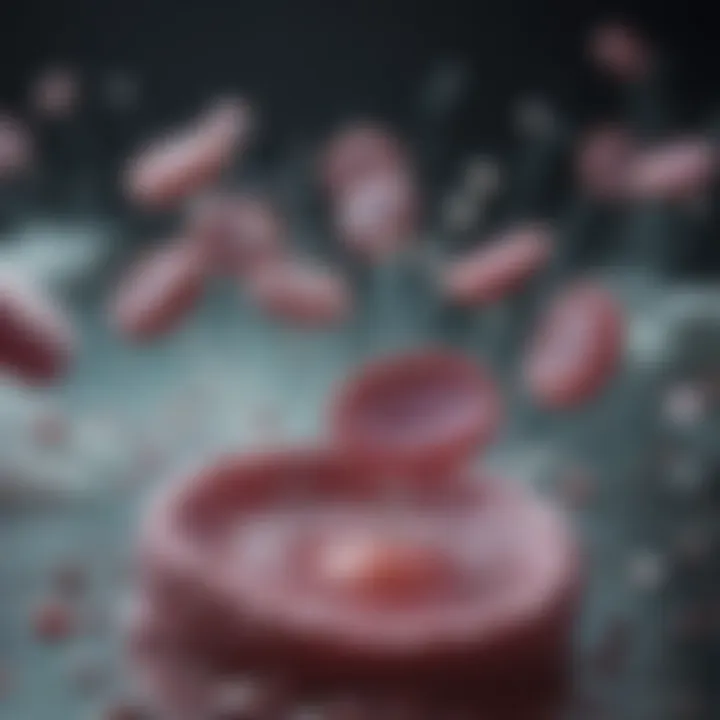
Primers and enzymes are essential components in the PCR process. Primers, typically 18-25 nucleotides long, are designed to be complementary to the target DNA sequence. They ensure that the amplification process is specific, allowing only the intended region of DNA to be duplicated.
The Taq polymerase enzyme, derived from the bacterium Thermus aquaticus, is equally important. It remains stable at high temperatures, which is crucial for the denaturation step. It indeed plays a pivotal role in synthesizing new DNA strands. Without specificity provided by primers and the robustness of Taq polymerase, PCR would become ineffective, much like trying to navigate a maze without a map.
Thermal Cycling Explained
Thermal cycling is the rhythmic dance of temperature variations that drives the PCR process. Each thermal cycle consists of three phases—denaturation, annealing, and extension—as previously stated.
This shifting of temperatures within the PCR thermocycler is pivotal. The precision allows the DNA to transition through different states, facilitating the essential reactions to take place. Generally, the cycling is set for 20-40 cycles, depending on the amount of starting material.
Remarkably, each cycle doubles the amount of target DNA, compounding your results
"In just a few hours, PCR can turn a minute amount of DNA into a volume that is sufficient for further analysis, making it an invaluable tool for science."
Understanding the biological mechanism of PCR offers invaluable insight into its functioning, making it a remarkable advancement in molecular biology. This knowledge not only aids in grasping its applications in contemporary science but also in appreciating the underlying principles driving this revolutionary technique.
Types of Pathogens Detected by PCR
The realm of Pathogens is vast, encompassing various microorganisms that can cause diseases in humans, animals, and plants. In the context of PCR testing, understanding the types of pathogens detected holds massive significance. This section will clarify how PCR serves as a frontline tool in identifying infections that range from the familiar cold virus to more severe bacterial infections. This capability is paramount for enhancing diagnostic accuracy, expediting treatment processes, and ultimately saving lives.
Bacterial Infections
Bacterial infections account for a significant portion of the maladies impacting public health. Traditional methods of identifying bacteria, such as culture techniques, can be slow and sometimes unreliable. PCR, however, slashes through this delay. The test can rapidly amplify specific DNA segments unique to pathogenic bacteria even from minuscule samples. This makes it particularly useful for detecting conditions such as tuberculosis or strep throat.
For example, when someone presents with symptoms of a serious infection, knowing immediately if Streptococcus pneumoniae is at play can help healthcare professionals make timely decisions on treatments.
"In diagnosing bacterial infections swiftly, PCR not only enhances patient outcomes but also helps control the spread of infectious diseases."
Moreover, the specificity of PCR means lesser chances of misdiagnosis. By targeting the DNA sequences unique to a particular bacterial species, healthcare providers can pinpoint the precise cause of an illness rather than simply making guesses based on symptoms alone.
Viral Pathogens
Viral infections follow closely behind bacterial infections in the toll they can take on health. Viruses, such as the influenza virus or the aforementioned SARS-CoV-2, require different methods of detection owing to their unique nature and replication processes. PCR serves to identify viral RNA rather than DNA, adapting its powerful amplification capabilities to these microscopic foes.
The speed at which PCR can deliver results is particularly crucial during viral outbreaks. When the Zika virus was proliferating, rapid PCR tests became central to managing and controlling infection spread, allowing public health officials to act swiftly and efficiently.
Testing for viral infections with PCR has proven to be a game-changer not only in clinical settings but also in epidemiological surveillance. As health crises unfold, real-time results enable data-driven decision-making alongside timely vaccination and healthcare resource allocation.
Fungal and Parasitic Detection
Fungi and parasites, while often overlooked, can wreak havoc in immunocompromised individuals or those with underlying conditions. Fungal infections like candidiasis or serious parasites such as Plasmodium (which causes malaria) often require more intricate detection methods. Here, PCR shines through again, providing a sensitive and rapid diagnostic tool.
The versatility of PCR allows it to amplify the genetic material of fungi and parasites, which is critical since cultures for these infections can take weeks to yield results. This speed not only aids in accurate diagnosis but also opens broader avenues for treatment when every moment counts.
In this arena, PCR tests can significantly improve clinical diagnostics. By enabling early and reliable detection, healthcare providers can tackle fungal and parasitic infections before they escalate, which is especially vital in vulnerable populations.
Applications of PCR Testing
The versatility of PCR testing is nothing short of remarkable. In today’s fast-paced world, where precision and speed are critical in diagnosing diseases, regulating environmental safety, and solving crimes, PCR plays a central role. The applications of this technology span across various fields, serving as a beacon for progress and innovation in molecular diagnostics. Understanding these applications is essential for appreciating the transformative impact of PCR on modern science and society.
Clinical Diagnostics
In clinical diagnostics, PCR testing is a game-changer. It allows for the rapid detection of pathogens and genetic disorders, which can be pivotal for timely treatment. For instance, in the context of infectious diseases, the speed at which PCR can identify a virus, such as SARS-CoV-2, often makes the difference between life and death. This method can detect minute amounts of viral RNA in a patient sample, which would be nearly impossible with traditional culture techniques.
Key benefits of PCR in clinical settings include:
- High Sensitivity: PCR can detect low levels of targeted DNA or RNA, making it very effective even in the early stages of infection.
- Rapid Results: The speed of PCR tests allows for quicker clinical decisions, leading to better patient management.
- Multiplexing Capability: PCR can be designed to detect multiple pathogens in a single test, which is particularly useful during outbreaks.
Moreover, PCR is not limited to infectious diseases. It is extensively used in oncology for identifying genetic mutations associated with cancer, allowing for personalized treatment approaches. The ability to analyze patient samples swiftly and accurately provides a powerful tool for clinical practitioners.
Forensic Science Utilization
The impact of PCR in forensic science is profound. This technology has revolutionized how evidence from crime scenes is analyzed. In scenarios where only a small biological sample—like a hair or a drop of blood— is available, PCR enables forensic experts to amplify DNA, making it possible to obtain a usable profile for comparison against databases.
Some aspects that highlight the significance of PCR in forensic applications include:
- Identification of Individuals: By analyzing genetic fingerprints, law enforcement can identify suspects or victims, playing a crucial role in criminal investigations.
- Cold Case Revivals: Older cases, which may have been unsolvable due to lack of evidence, can be revisited as technology improves and more advanced PCR methods emerge.
- Exonerating the Innocent: PCR has also exonerated individuals wrongly convicted, showcasing its importance in the justice system.
These capabilities underline why PCR is a cornerstone in forensic laboratories. The ability to deliver quick and accurate results makes it an indispensable tool for investigators.
Environmental Testing Applications
PCR technology is not confined to human health. Its application in environmental testing addresses the pressing need for monitoring microbial contamination in water, soil, and food products. By utilizing PCR, environmental scientists can detect harmful pathogens and toxins that may pose risks to public health.
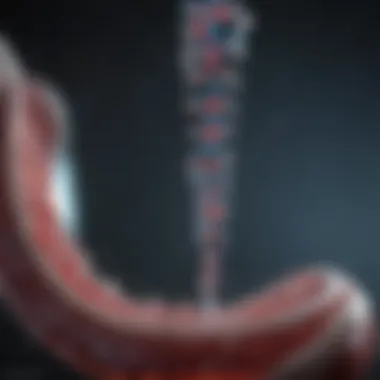
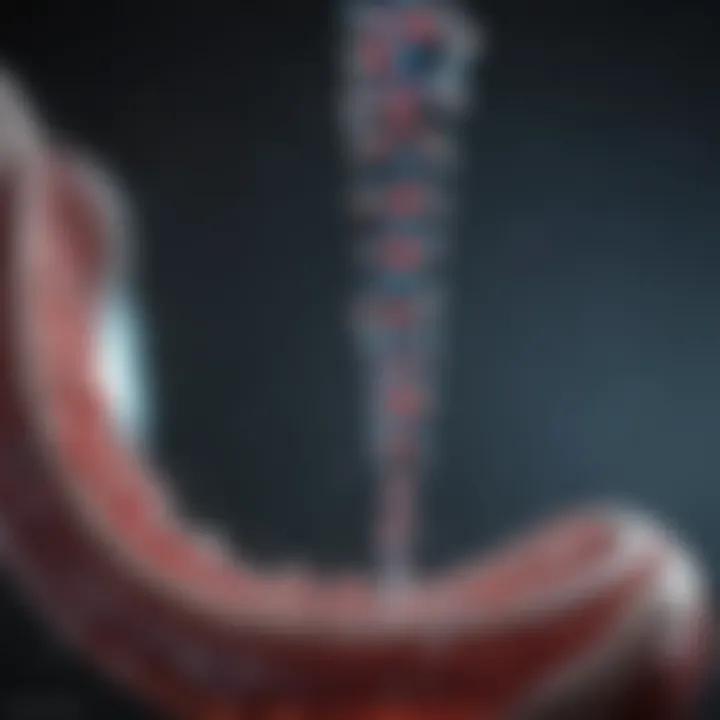
Important highlights of PCR in environmental contexts include:
- Pathogen Detection: PCR rapidly identifies bacteria and viruses in water supplies, crucial for drinking water safety.
- Environmental Monitoring: With the rise of concerns regarding biosecurity and ecology, PCR assists in monitoring biodiversity by detecting species present in a given area, shedding light on ecosystem health.
- Food Safety: Detection of foodborne pathogens before they can cause outbreaks is another vital application, safeguarding consumer health.
Overall, the ability to detect a variety of pathogens and genetic materials in environmental samples highlights PCR's essential role in ensuring the safety and health of our ecosystems.
"PCR is not just a scientific technique; it's a bridge connecting the laboratory to real-world applications that impact lives."
In summary, the applications of PCR testing are vast and varied. It goes beyond mere diagnostics and probes into forensic insight and environmental safety, proving itself a critical tool across multiple domains. As science continues to advance, the full potential of PCR testing continues to unfold, shaping the future of diagnostics and safety in numerous fields.
Interpreting PCR Test Results
Interpreting the results of a PCR test is critical, as it can have profound implications for diagnosis, treatment, and disease management. Accurate interpretation requires a solid grasp of the nuances behind sensitivity and specificity, as well as an understanding of the potential for false positives and negatives. Without this knowledge, the results could lead to misinformed decisions.
Understanding Sensitivity and Specificity
Sensitivity and specificity are two foundational concepts in interpreting PCR test results. Sensitivity refers to the test's ability to correctly identify true positives. In simpler terms, if a test has high sensitivity, it means that individuals with the condition are less likely to receive a negative result. This is crucial in a clinical setting where missing a diagnosis might lead to progression of disease.
For example, if a PCR test for a viral infection has a sensitivity of 95%, it truly identifies 95% of those who are infected. If we consider a population of 100 infected individuals, only five of them would erroneously test negative. This characteristic is particularly valuable in early diagnosis of infectious diseases.
On the other hand, specificity is all about true negatives. It measures the ability of the test to correctly identify those without the disease, minimizing the incidence of false positives. A test with high specificity ensures that individuals who do not have the condition are correctly identified as negative.
Let’s say a PCR assay for a bacterial infection boasts 90% specificity. In a healthy group of 100 individuals, only 10 would get identified as positive, which is acceptable depending on the context of the disease prevalence in the population. Balancing these two elements is fundamental. In brief:
- High Sensitivity: Valuable for ruling out disease; fewer false negatives.
- High Specificity: Essential for confirming disease; fewer false positives.
Impact of False Positives and Negatives
The implications of false results in PCR testing cannot be overstated, as they can lead to a cascade of unintended consequences. A false positive result occurs when a person tests positive for a pathogen when they do not actually have it. This can provoke unnecessary anxiety, potential misuse of healthcare resources, and even inappropriate treatments. For instance, a patient may start an antiviral treatment based on a misdiagnosis, which could expose them to unnecessary side effects.
Conversely, a false negative result can be even more dangerous. This happens when an infected person receives a negative test result, leading them to believe they are healthy. Continuing to interact socially or neglecting treatment can allow the disease to spread, affecting not only the individual but the wider community as well.
Some common scenarios affected by false results include:
- Public Health Responses: A spike in false positives may lead health officials to implement containment measures unnecessarily, affecting individuals' lives and economies.
- Clinical Decision-Making: Clinicians may decide on treatment plans based on inaccurate information, complicating the patient's health journey.
- Patient Confidence and Behavior: Repeated misdiagnosis can erode trust in healthcare systems, prompting some to avoid seeking medical help altogether.
Accurate interpretation of PCR results is paramount as it ensures effective and appropriate healthcare responses, ultimately saving lives.
The complexity of interpreting PCR results calls for practitioners to consider the broader context, including patient history, symptoms, and prevalence of disease in their local population. Awareness of the test’s limitations is key to providing a holistic view, ensuring patients receive informed, effective care.
Limitations of the PCR Test
The Polymerase Chain Reaction (PCR) test, while groundbreaking in the field of molecular diagnostics, is not without its challenges and limitations. Understanding these limitations is crucial for researchers, clinicians, and educators alike, as they navigate the intricacies of PCR testing in diverse applications. The constraints of the PCR process can impact test accuracy, reliability, and ultimately, healthcare decisions. This section explores some of the primary limitations associated with PCR testing, particularly technical constraints and issues related to sample quality and handling.
Technical Constraints
One of the foremost limitations of PCR is its technical constraints. The PCR process hinges on several critical parameters that, if not optimized, can lead to inaccurate results. Key technical constraints include:
- Contamination Risk: The high sensitivity of PCR means that even minute amounts of DNA can trigger amplification. This sensitivity, while a strength, can also be a double-edged sword; cross-contamination from other samples, laboratory equipment, or even aerosolized particles can lead to false positives. A common scenario is an amplified result reeking of contamination, where minute fragments of DNA from unrelated sources spoil the assay.
- Reaction Conditions: Successful PCR relies on specific temperatures, buffer compositions, and enzyme activities. Deviations in temperature can greatly influence the efficiency of the reaction, leading to suboptimal results. Additionally, variations in pH or ionic strength can affect enzyme function, which may compromise the quality of the amplification. The delicate balancing act that is required makes standardization critical.
- Limited Specificity: Although PCR offers high sensitivity, it may lack specificity, particularly if the primers used are not well designed. Primers are critical for targeting specific genetic sequences, but flawed primer design may lead to non-target amplification. This can be problematic in diagnostic tests, where distinguishing pathogens from the host’s DNA is crucial.
Overall, these technical limitations serve as a reminder that while PCR is a powerful tool, meticulous attention to detail during the assay development and execution phases is imperative.
Sample Quality and Handling Issues
The quality of the sample being tested plays a pivotal role in the overall success of the PCR test. Below are some prevalent sample-related issues that can hinder accuracy:
- Degradation of Nucleic Acids: Samples collected poorly or stored inappropriately may result in the degradation of nucleic acids, diminishing the amount of usable DNA or RNA in the sample. Factors such as temperature fluctuations can severely affect the integrity of the genetic material. For example, a sample that has been left at room temperature for too long may yield unreliable PCR results due to its degraded state.
- Inhibition Factors: Certain substances present in biological samples, including proteins, phenolic compounds, and salts can inhibit the PCR reaction. These inhibitors can bind to the DNA polymerase or interfere with the primers, preventing efficient amplification. For instance, samples collected from complex matrices such as fecal material or soil may introduce unexpected inhibitors that impede the PCR process.
- Collection Technique: The methodology used for sample collection is also a key factor. Proper techniques must be implemented to minimize contamination and ensure that the sample accurately represents the target pathogen. Missteps during this process can lead to biased results, rendering the PCR test ineffective.
"Errors in sample collection or handling can significantly skew the results of PCR tests, leading to misguided conclusions in diagnostics."
In summary, while PCR technology has revolutionized diagnostics, understanding its limitations, particularly concerning technical constraints and sample quality, is paramount. These factors must be addressed to enhance the reliability and accuracy of PCR as a diagnostic tool.
Technological Advancements in PCR
The landscape of Polymerase Chain Reaction (PCR) has seen remarkable changes over the past few decades. These advancements not only enhance the accuracy of the test but also expand its applications in various fields such as healthcare, forensics and environmental science. Understanding these technological strides is vital as they fundamentally improve the efficiency and reliability of PCR testing. Here, we delve into two prominent developments that have reshaped how PCR is conducted: Real-Time PCR and Digital PCR.
Real-Time PCR Developments
Real-Time PCR, also known as quantitative PCR (qPCR), has emerged as a game-changer in the realm of molecular diagnostics. This method allows for the quantification of DNA as it is amplified, providing immediate and precise data. Here are some of the specific benefits of Real-Time PCR:
- Real-Time Results: Unlike traditional PCR, which concludes after the amplification process, Real-Time PCR continuously monitors the progress, allowing for quick insights into the quantity of the target DNA.
- High Sensitivity: This technology can detect even small amounts of DNA, making it exceedingly valuable for early diagnosis of infectious diseases and cancer.
- Multiplexing Capability: Real-Time PCR enables the simultaneous detection of multiple targets in a single reaction, reducing sample usage and time.
The importance of Real-Time PCR is not just technical; it also has substantial implications for patient care. Clinicians can quickly adjust treatment protocols based on real-time data, ultimately leading to better patient outcomes. Furthermore, its usage in monitoring viral loads in patients with contagious diseases like HIV or during a pandemic, like COVID-19, highlights its critical role in public health interventions.
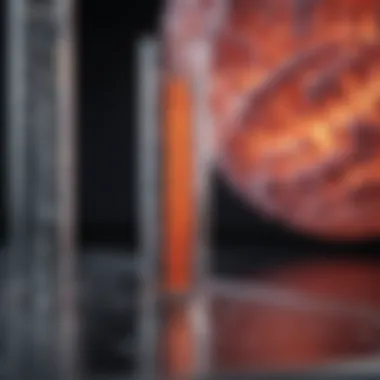
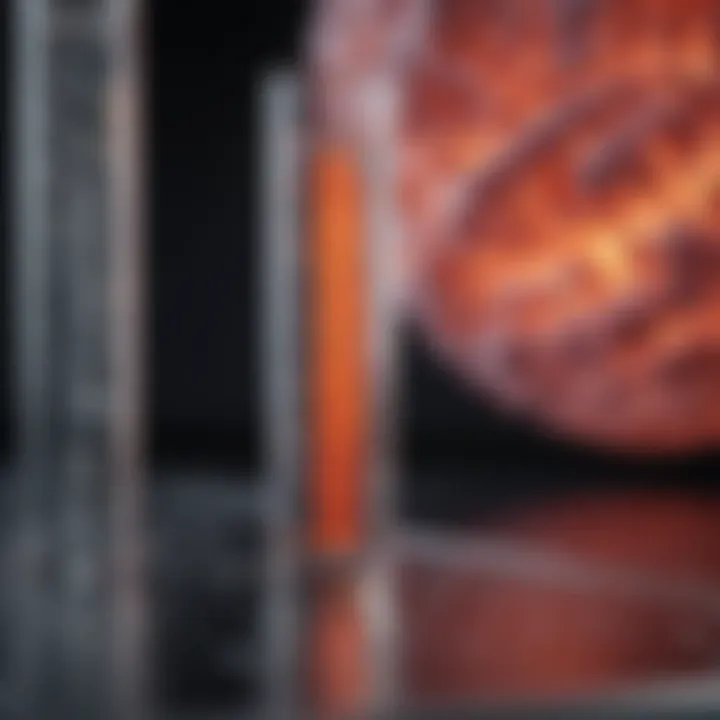
Digital PCR Innovations
Digital PCR represents another significant leap in PCR technology. It offers a unique and distinct approach to nucleic acid quantification unlike any traditional method. Here are some vital considerations regarding Digital PCR:
- Absolute Quantification: Digital PCR provides precise quantification of DNA without the need for standard curves, making it incredibly reliable for low-abundance targets.
- High Precision: Utilizing microfluidic techniques, Digital PCR partitions the sample into thousands of separate reactions, allowing for increased sensitivity and accuracy in quantification.
- Robust against Inhibition: This method is more resilient to the inhibitory effects often encountered in PCR, thus enhancing reliability in samples that may have contaminants, such as those from environmental sources.
These innovative approaches are not just beneficial; they present new opportunities for tackling pressing issues in genetic research and clinical applications. For example, Digital PCR can be pivotal in cancer research, where detecting minimal residual disease can dictate treatment decisions. It offers key advancements that align with personalized medicine's goals, illustrating how technology and health science interconnect.
"Technological advancements in PCR have empowered researchers and clinicians alike, elevating diagnostic accuracy and paving the way for groundbreaking discoveries in genomics."
Overall, as we navigate through the evolving landscape of PCR technology, understanding these advancements can lead one to appreciate their profound impact on medical diagnostics and beyond.
Future Perspectives on PCR Testing
As we look ahead, the field of PCR testing stands at an exciting crossroads, marked by profound advancements and growing integration into various domains of healthcare and research. The importance of exploring these future perspectives cannot be overstated; it not only highlights the evolving capabilities of PCR tests but also provides a glimpse into how they will shape diagnostic processes and patient care in the years to come.
Emerging Trends in Molecular Diagnostics
The landscape of molecular diagnostics is rapidly changing, with PCR tests at the forefront. One key trend is the rise of point-of-care testing. This approach minimizes the time between sample collection and result generation, offering the potential for immediate patient management. Instead of waiting days for lab results, healthcare providers could get results on the spot, which can drastically improve clinical decision-making.
Moreover, the development of high-throughput technologies is revolutionizing how tests are conducted. By enabling the simultaneous analysis of multiple samples, labs can process larger volumes of data efficiently. This not only increases the speed of testing but also boosts the throughput capabilities of clinical laboratories. Efficiency like this is crucial, especially during times of public health emergencies.
Another trend is the use of multiplex PCR, which allows for the detection of several pathogens in a single reaction. This is particularly beneficial in diagnosing co-infections, where multiple pathogens may be present and could complicate treatment if not identified early.
"The future of PCR testing is not just about speed and efficiency; it is equally about precision and adaptability in diagnosing diseases."
Integration with Next-Generation Sequencing
The synergy between PCR testing and next-generation sequencing (NGS) is poised to unlock new possibilities in molecular diagnostics. While PCR allows for targeted amplification of specific DNA sequences, NGS provides a broader view by enabling the sequencing of entire genomes or targeted regions. This combination creates a powerful tool for researchers and clinicians alike.
Integrating PCR with NGS can significantly enhance both the sensitivity and specificity of diagnostic tests. For example, by first amplifying regions of interest using PCR, followed by sequencing, clinicians can identify mutations or variants in pathogens that may influence treatment strategies. This is particularly important in fields such as oncology, where understanding genetic mutations can dictate therapeutic choices.
Furthermore, this integration facilitates personalized medicine, where treatments can be tailored to an individual’s genetic makeup, optimizing therapeutic effectiveness and minimizing adverse effects.
Ethical Considerations in PCR Testing
The rise of PCR testing has not only transformed the landscape of molecular diagnostics but has also raised a slew of ethical questions that can't be brushed aside. This section aims to probe into the vital ethical considerations surrounding PCR testing, particularly concerning privacy and access issues. Understanding these dimensions is crucial for both the scientific community and society at large.
Privacy Concerns and Data Management
The swiftness with which PCR tests collect and analyze genetic data poses significant challenges to privacy. When a sample is taken, it is often accompanied by a considerable amount of personal information. This creates a potential gold mine for misuse if not managed properly. It's one thing to catch a virus; it’s another to have one's genetic information floating around.
PCR testing generates results that can reveal susceptibilities to various conditions. Therefore, the implications of handling such sensitive data warrant careful consideration. Laboratories and testing facilities must ensure robust data protection measures.
In particular, adherence to guidelines—like the Health Insurance Portability and Accountability Act (HIPAA) in the U.S.—is critical for safeguarding patient information. Clear policies and transparency on how data will be utilized or stored must be communicated to individuals getting tested. With the technology evolving rapidly, it's a fight to ensure confidentiality in an age where data breaches are distressingly common.
"It's essential that consent isn't just a formality but a fundamental part of the testing process."
Equity and Access to Testing
Another ethical layer relates to the equity of access to PCR testing. While PCR tests have rapidly become pivotal in diagnosing diseases like COVID-19, disparities in access remain glaring. It's noteworthy how socioeconomic status and geographic location can dictate who gets tested and who doesn’t. Individuals in remote areas or belonging to lower income brackets may struggle to access timely testing, leaving them at a disadvantage.
To foster equitable healthcare, stakeholders must address these stark inequalities. Initiatives to fund free or low-cost testing centers can go a long way in bridging gaps.
Consider implementing mobile testing units targeted at underserved communities—similar to how some organizations operate free clinics. These efforts are not just commendable; they are imperative.
Key Points on Ethical Considerations in PCR Testing:
- Data Privacy: Secure handling of sensitive genetic information is vital to maintain trust.
- Regulatory Compliance: Adhere to strict national and international laws governing personal health data.
- Accessibility: Ensure equitable distribution of testing facilities and resources, especially for marginalized communities.
- Community Engagement: Foster an open dialogue between testing providers and the public to build trust and transparency.
In summary, ethical considerations in PCR testing are multi-faceted and critical. As we advance in our diagnostic capabilities, we must ensure that this progress is inclusive, responsible, and ethically sound.
Culmination
The significance of a well-rounded conclusion in this article cannot be overstated. While many readers may glance through sections, it is essential to recognize that the conclusion serves as the final bookmark, enabling individuals to distill the myriad insights the article unfolds. In this case, it synthesizes crucial elements surrounding PCR testing, its application across a variety of fields, and the associated ethical considerations necessary in the present climate.
Summarizing Key Insights
In reviewing the points discussed, a few key insights stand out:
- PCR's Fundamental Role: The Polymerase Chain Reaction has revolutionized molecular diagnostics, offering rapid, sensitive detection of pathogens.
- Diverse Applications: Whether applied in clinical settings or forensic analysis, the flexibility of PCR technology is remarkable.
- Technological Evolution: As PCR continues to evolve with real-time and digital advancements, it opens doors to more accurate and comprehensive analysis.
- Ethical Dimensions: The handling of genetic data raises pertinent privacy and equity questions that society must grapple with.
A summation of these insights aids in reinforcing why PCR cannot be overlooked in medical and scientific realms.
Implications for Future Research
Moving forward, several avenues for research warrant attention:
- Integration with Other Technologies: Examining how PCR can synergize with next-generation sequencing could yield profound implications in diagnostics.
- Addressing Limitations: Delving deeper into the technical constraints and sample handling challenges can pave the way for improved methodologies.
- Ethical Frameworks: Developing robust ethical standards around data management and access can ensure the benefits of PCR extend equitably across populations.
- Expanding Beyond Infectious Diseases: Looking into applications of PCR for non-infectious conditions, like cancer detection and genetic disorders, could further broaden its impact.
As we immerse ourselves into these considerations, it's clear that the journey of understanding the implications of PCR testing isn’t merely about the science—it’s also about navigating the intricate landscape of ethics and technology together. Greater commitment to research in these areas will only enhance our capabilities in leveraging PCR for the greater good.