Exploring Human Brain Imaging Techniques and Insights
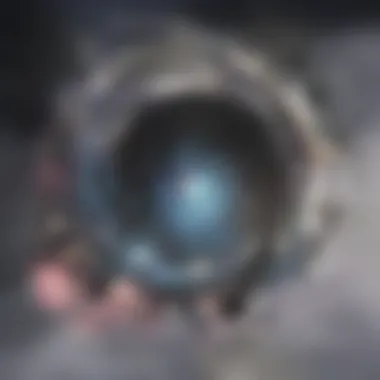
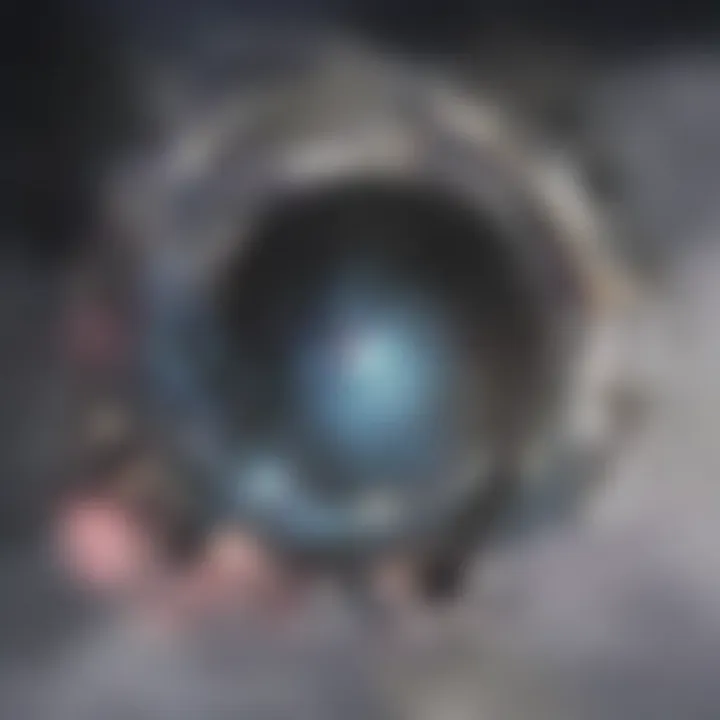
Intro
The human brain stands as one of the most complex structures in existence. Understanding it requires precision and detail, often best captured through advanced imaging techniques. This article aims to provide a comprehensive overview of the various methodologies used in brain imaging. It also explores the implications of technological advancements, the benefits gained, and the ethical considerations surrounding this field of research. Knowledge of these techniques not only enhances our understanding of brain structures and functions but also opens doors for future innovations in medical science.
Research Overview
Summary of Key Findings
Brain imaging techniques have evolved significantly in recent years. Techniques such as Magnetic Resonance Imaging (MRI) and Positron Emission Tomography (PET) offer remarkable insights into brain activity and structure. Findings indicate that different modalities can complement each other. For instance, MRI excels at providing structural images, while PET offers metabolic information, allowing researchers to piece together a more complete picture of brain state and function.
- MRI provides detailed images of brain anatomy.
- PET reveals patterns of brain activity through metabolic processes.
- Functional MRI (fMRI) helps understand real-time brain function in response to tasks.
Significance of Research
Understanding the human brain through these imaging techniques has profound implications for various fields. It aids in diagnosing neurological disorders, tracking disease progression, and evaluating treatment effectiveness. The ability to visualize brain activity in real-time allows for groundbreaking studies on cognition, emotion, and behavior. As these technologies improve, the potential to uncover new truths about the brain expands.
"The brain is a complicated organ, but with advanced imaging we can start to decipher its many secrets."
Methodology
Research Design
The research design for studying brain imaging techniques involves a combination of clinical and experimental approaches. Various studies may employ cross-sectional designs to capture a snapshot of brain activity or longitudinal designs to observe changes over time. This flexibility enables researchers to tailor their studies to specific questions, be it examining the effects of a particular therapy or understanding the neural basis of behavior.
Data Collection Methods
Data collection is vital in brain imaging studies. Various techniques are employed to ensure reliable and comprehensive data:
- MRI Scans: Utilize magnetic fields and radio waves to generate high-resolution images.
- PET Scans: Involve injecting a radioactive tracer to measure metabolic activity.
- fMRI: Measures brain activity by detecting changes in blood flow using magnetic fields.
These methods, while distinct, often work best in conjunction. A multimodal approach can yield richer data, offering insights that single-modality studies might miss.
Prelims to Brain Imaging
Brain imaging is an area of study that holds immense importance in neurosciences, medicine, and even psychology. It has the power to considerably enhance our understanding of the human brain. Brain imaging techniques allow for the visualization of both the structure and function of the brain, providing critical insights into various neurological and psychological conditions.
These imaging techniques are essential for diagnosing diseases, guiding treatment decisions, and advancing research. The ability to observe the brain in real-time paves the way for innovative methods to comprehend complexities associated with brain functionality. Additionally, the continuous improvement of imaging technologies plays a pivotal role in revealing new layers of understanding about cognitive processes and brain mappings.
Defining Brain Imaging
Brain imaging refers to the range of techniques used to visualize the structure and function of the brain. This includes methods like Computed Tomography (CT), Magnetic Resonance Imaging (MRI), and functional MRI (fMRI). These scans help capture images that reflect the brain's composition, blood flow, and neural activity.
The primary goal of brain imaging is to provide a clearer perspective on how the brain operates in both healthy and diseased states. Techniques vary in their approach, with some focusing on the anatomical aspects while others pay closer attention to physiological functions. As a whole, brain imaging serves as a gateway to unlocking knowledge about human cognition, behavior, and neurological disorders.
Historical Context and Evolution
The development of brain imaging techniques has progressed significantly over the past several decades. Early methods, such as X-rays, provided limited information due to their inability to capture soft tissue clearly. The introduction of CT scans in the 1970s marked a turning point, enabling a more detailed examination of the brain's structure.
Later, the invention of MRI technology brought a new era of imaging, offering improved contrast and more accurate resolution without exposing patients to ionizing radiation. The emergence of functional imaging, particularly fMRI and PET scans, further enriched our understanding by allowing us to visualize brain activity in response to various stimuli.
Today, brain imaging is crucial in both clinical and research settings. It facilitates diagnosis and informs treatment while also driving findings in cognitive neuroscience and psychology.
Types of Brain Imaging Techniques
Understanding the types of brain imaging techniques is crucial for exploring the complexities of the human brain. Each technique offers unique insights into brain structure and function. By employing various methodologies, researchers and clinicians can obtain valuable data that aids in diagnosis, treatment, and further research. This section discusses the most prominent techniques and highlights their specific characteristics, benefits, and limitations.
Structural Imaging
Structural imaging techniques provide detailed information about the anatomy of the brain. They allow for the visualization of brain structures, helping professionals identify abnormalities such as tumors or lesions. The most commonly used structural imaging techniques are Computed Tomography (CT) and Magnetic Resonance Imaging (MRI).
Computed Tomography (CT)
Computed Tomography, or CT, is a widely used medical imaging technique that creates detailed cross-sectional images of the brain. It utilizes X-rays and advanced computer processing to generate comprehensive views. One of the key characteristics of CT is its speed; it can produce images quickly, making it a go-to option for emergency situations.
A unique feature of CT is its ability to visualize bones and dense structures clearly, which can be particularly useful when assessing head injuries or fractures. However, CT also has disadvantages, such as exposure to ionizing radiation, which could limit its frequent use in certain populations, like children.
Magnetic Resonance Imaging (MRI)
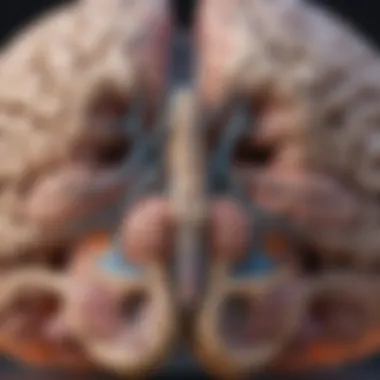
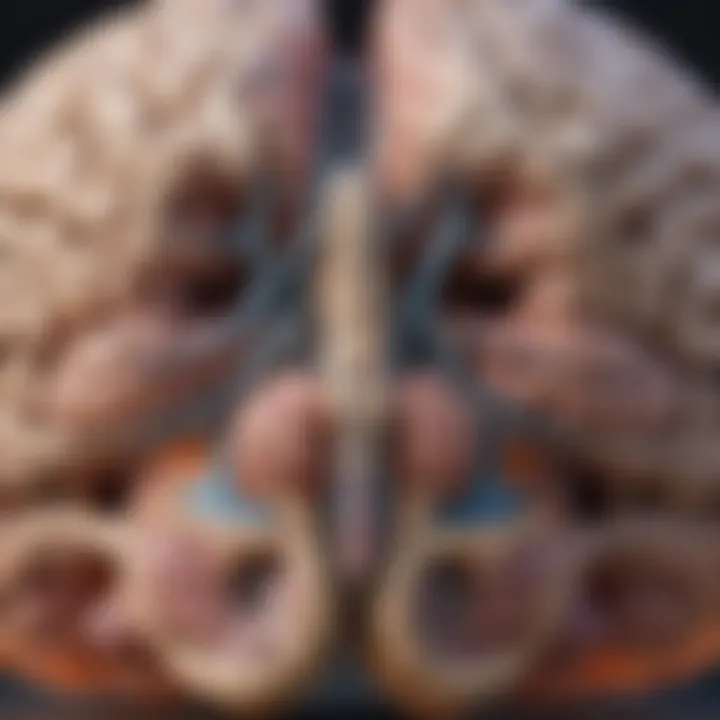
Magnetic Resonance Imaging, or MRI, uses powerful magnets and radio waves to create highly detailed images of the brain. This technique excels in soft tissue visualization, making it invaluable for examining brain injuries, tumors, and conditions like multiple sclerosis. A key characteristic of MRI is its ability to create images without exposing patients to radiation. This aspect makes it a safer option for repeated use.
MRI has several unique features, including the ability to provide distinct images based on tissue type. However, the longer imaging times and potential discomfort due to the enclosed space of the MRI machine can be challenging for some patients.
Functional Imaging
Functional imaging techniques assess the brain's activity. They provide insights into how different areas of the brain function and respond during various tasks. Functional MRI (fMRI) and Positron Emission Tomography (PET) are among the leading methods in this category.
Functional MRI (fMRI)
Functional MRI, or fMRI, measures changes in blood flow to different regions of the brain while a person performs tasks or experiences stimuli. This technique captures the dynamic nature of brain activity with excellent spatial resolution. A significant advantage of fMRI is that it does not involve radiation, making it safer for participants.
The unique feature of fMRI is the blood-oxygen-level-dependent (BOLD) signal, which reflects neural activity. However, fMRI can have limitations, like sensitivity to motion, which may affect the quality of images if a participant moves during the scan.
Positron Emission Tomography (PET)
Positron Emission Tomography, or PET, utilizes radioactive tracers to visualize metabolic processes in the brain. This technique provides valuable information regarding the brain's functional activity and is particularly useful in oncology, neurology, and psychiatry. A key characteristic of PET is its ability to detect changes at a cellular level, offering insights that structural imaging cannot.
The unique feature of PET includes its ability to visualize receptor binding and neurotransmitter activity. However, one disadvantage is the exposure to radiation from the tracers, which poses considerations for long-term studies.
Electrophysiological Imaging
Electrophysiological imaging techniques measure electrical activity in the brain. They provide real-time data on brain functions, useful for diagnosing various neurological disorders. Electroencephalography (EEG) and Magnetoencephalography (MEG) are prominent methods in this category.
Electroencephalography (EEG)
Electroencephalography, or EEG, records electrical activity along the scalp. EEG is essential for diagnosing conditions like epilepsy and sleep disorders. A primary advantage of EEG is its high temporal resolution, allowing researchers to capture rapid changes in brain activity.
A distinctive feature of EEG is its portability and affordability, making it accessible for various clinical settings. However, EEG has limitations in spatial resolution, which might hinder pinpointing the exact location of brain activity.
Magnetoencephalography (MEG)
Magnetoencephalography, or MEG, measures the magnetic fields produced by neural activity. This technique provides both high temporal and spatial resolution. MEG is particularly valuable for mapping brain functions before surgical interventions. A key characteristic of MEG is its ability to track brain activity in real time.
The unique feature of MEG is its non-invasive nature, which allows for safe and repeatable studies. However, similar to EEG, the equipment can be costly and requires specialized facilities and training for accurate interpretation.
Principles of Imaging Technologies
Imaging technology plays a pivotal role in our understanding of the human brain. It encompasses various physics-based principles and signal processing techniques that allow us to visualize and interpret the intricate structures and functionalities of neural tissues. The significance of these principles cannot be overstated, as they form the foundation for many modern brain imaging techniques, enabling advancements in both clinical and research settings.
Physics Behind Imaging Techniques
The physics behind imaging techniques is crucial for the accurate representation of brain anatomy and function. Each imaging modality relies on distinct physical principles that govern how images are generated and interpreted.
- Computed Tomography (CT): This technique uses X-rays to create cross-sectional images of the brain. By rotating around the patient's head, the CT scanner captures multiple images from different angles. These images are processed using complex algorithms to reconstruct a detailed three-dimensional view of brain structures. The physics of radiographic attenuation is central to CT imaging, where different tissues absorb X-rays at varying degrees. Dense materials, such as bone, appear brighter, while softer tissues, such as gray matter, show different shades of gray.
- Magnetic Resonance Imaging (MRI): MRI operates on principles of nuclear magnetic resonance. It employs strong magnetic fields and radio waves to excite hydrogen atoms in the body, particularly in water-rich tissues like the brain. When these atoms return to their equilibrium state, they emit signals that are processed to form detailed images of brain structures. The high spatial resolution of MRI is attributed to the magnetic properties of different tissues, providing sharp contrast between gray matter, white matter, and cerebrospinal fluid.
- Functional MRI (fMRI): A specialized form of MRI, fMRI measures brain activity by detecting changes in blood flow. It leverages the principle of blood-oxygen-level-dependent (BOLD) contrast. Areas with increased activity consume more oxygen and thus receive a more significant blood supply, resulting in identifiable signal changes. This enables researchers to observe brain activation patterns during specific tasks or stimuli, granting insights into cognitive processes.
Understanding these physical principles enriches our comprehension of brain structure and function, ensuring that imaging results are interpreted correctly.
Signal Processing in Brain Imaging
Signal processing constitutes a critical component in brain imaging. It involves the manipulation and analysis of the data collected by imaging devices. The raw data obtained from imaging technologies often require substantial processing to highlight significant features and remove artifacts that may obscure true signals.
- Fourier Transform: This mathematical tool is extensively used in MRI. It converts time-domain signals into frequency-domain data, allowing visualization of the spatial distribution of signals in the brain. By applying Fourier transforms, clinicians and researchers can enhance image resolution and contrast.
- Image Reconstruction Algorithms: Advanced algorithms transform raw imaging data into comprehensible visualizations. Techniques like iterative reconstruction improve image quality while reducing noise. These algorithms account for various distortions encountered during imaging, ensuring that the final product is as accurate as possible.
- Machine Learning Applications: Recently, machine learning has emerged as a powerful signal-processing tool in brain imaging. Algorithms can analyze imaging data to identify patterns associated with various brain conditions, such as tumors or neurodegenerative diseases. The use of deep learning to recognize complex features aids in diagnostic accuracy and research development.
In summary, the principles of physics and signal processing in imaging technologies play an indispensable role in enhancing our understanding of the human brain. By grasping these concepts, we can appreciate the intricate relationship between the technology and the rich data it generates, ultimately leading to improved clinical practices and innovative research advancements.
Applications of Brain Imaging
The utility of brain imaging extends far beyond simple visuals of our brain's structure. This section encompasses important areas where imaging techniques are pivotal. Here, we explore applications in clinical diagnostics, research and development, and post-surgical monitoring, offering insights into the benefits and relevance of these techniques.
Clinical Diagnostics
Brain Tumors
Brain tumors present a specific challenge in diagnostics. Imaging plays a critical role in identifying and characterizing these tumors. Advanced imaging technologies, like MRI and CT scans, can provide detailed information about tumor size, location, and even type. This capability enhances the overall treatment planning.
One key characteristic of brain tumors is their ability to present varied appearances on imaging scans. Tumors can differ in density, enhancing characteristics, and surrounding edema. Understanding these distinctions is important, as they can indicate the tumor's behavior and aggressiveness. This specificity emphasizes why brain imaging is a beneficial approach in clinical settings.
The unique feature of using imaging for brain tumors is its ability to monitor changes over time. Doctors can track tumor growth or response to treatment effectively. However, there are disadvantages; not all tumors are detectable by imaging alone, and sometimes, results may be misleading due to overlapping features with other conditions.
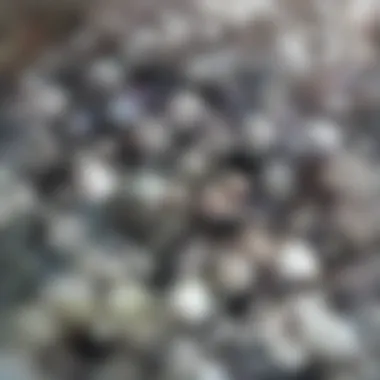
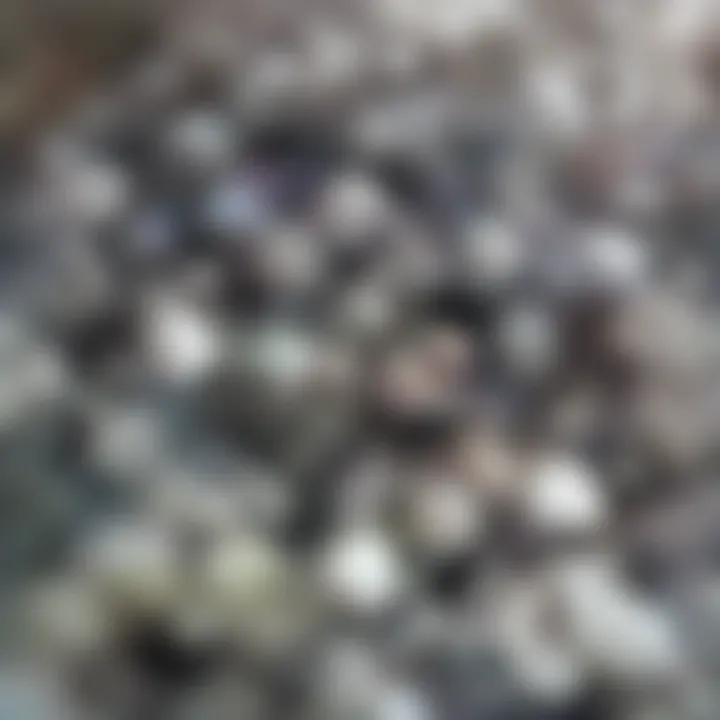
Neurodegenerative Diseases
Neurodegenerative diseases are a critical area where brain imaging is invaluable. Conditions such as Alzheimer's and Parkinson’s disease require precise identification and monitoring. Imaging techniques can reveal patterns of brain atrophy and metabolic slowdowns, which contribute significantly to diagnosis and treatment strategies.
A key characteristic of these diseases is their gradual progression. This allows imaging to capture subtle changes in brain structure over time. This aspect makes it a preferred choice for ongoing research and clinical diagnosis, as it assists in understanding disease progression and patient prognosis.
The unique aspect of studying neurodegenerative diseases through imaging lies in its ability to differentiate between various types of dementia. This diagnostic richness helps in tailoring treatments specifically for each condition. Challenges include variations in imaging results among patients and the need for comprehensive interpretation by specialists, as not all neurodegenerative changes are identifiable through standard imaging.
Research and Development
Cognitive Neuroscience
Cognitive neuroscience explores the link between brain functions and cognitive processes. Imaging techniques allow researchers to visualize brain activity in real-time. This aspect is crucial for understanding how different areas of the brain contribute to memory, language, and decision-making.
The key feature of cognitive neuroscience in this context is the capability to correlate brain imaging results with behavioral data. This interrelation fosters deeper insights into complex cognitive phenomena, making brain imaging an essential tool in this field.
The unique advantage of using imaging techniques here is their non-invasive nature. This allows for repeated studies on the same subjects, leading to more reliable data. However, challenges remain, such as the variability in individual brain anatomies and activity levels, which may complicate interpretations of cognitive tasks.
Psychiatric Research
Psychiatric research leverages brain imaging to delve into the complexities of mental health conditions. Disorders like depression, schizophrenia, and bipolar disorder can manifest unique patterns observable through imaging. Understanding these patterns aids in understanding the biological underpinnings of these conditions.
A key consideration in psychiatric research is the heterogeneity of mental illnesses. Each condition can present differently in terms of brain activity and structure. This variability makes brain imaging a popular tool as it allows for the exploration of individualized treatment approaches.
The unique feature of this application is how imaging can assist in correlating symptoms with neural circuitry. This can lead to more targeted therapies. There are disadvantages as well; interpreting images in the context of psychiatric disorders can be complex due to fluctuating symptoms and often requires specialized knowledge.
Post-Surgical Monitoring
Post-surgical monitoring is another crucial application of brain imaging. After interventions such as tumor resections or epilepsy surgeries, imaging helps ensure that the surgical objectives were achieved and identifies any possible complications. Using tools like MRI and CT scans immediately post-surgery provides invaluable information.
Case Studies of Brain Imaging
Case studies in brain imaging provide crucial insights that bridge the gap between theory and practice in neuroscience. They showcase how imaging techniques apply in real-world research and medical settings. These studies often highlight the applications of various imaging modalities, revealing their strengths and limitations. This section emphasizes the significance of case studies in demonstrating the impact of imaging on understanding the human brain.
Successful Use in Research Studies
Research studies utilizing brain imaging techniques have offered profound advancements in our understanding of neurological and psychological conditions. For instance, functional Magnetic Resonance Imaging (fMRI) has facilitated the mapping of brain activity in response to specific tasks, such as language processing or memory retrieval. One notable case study involved tracking brain activation patterns in individuals performing a visual memory task. The results identified regions in the occipital and frontal lobe that were significantly engaged during this task, enhancing our grasp of memory mechanisms.
Moreover, brain imaging studies have played a pivotal role in cognitive neuroscience. They have explored the neural basis of decision-making, perception, and emotional responses. For example, research examining how the brain responds to complex social scenarios has illuminated the involvement of areas like the prefrontal cortex and amygdala, which are crucial for social cognition.
These successful case studies not only advance academic understanding but also present promising data for therapeutic interventions targeted at various conditions. Whether it is optimizing treatment plans for anxiety disorders or developing training programs for cognitive enhancement, brain imaging lays foundational knowledge for medical advancements.
Impact on Clinical Practices
The impact of brain imaging on clinical practices cannot be understated. Neuroimaging techniques have transformed how clinicians diagnose and manage brain-related disorders. Case studies illustrate practical applications, such as the identification of brain tumors through Magnetic Resonance Imaging (MRI). Physicians can assess tumor size, location, and effects on surrounding neural structures. This information is critical in devising surgical strategies and understanding potential risks involved.
In the context of neurodegenerative diseases, imaging studies have aided in early diagnosis, improving patient outcomes through timely intervention. For instance, Positron Emission Tomography (PET) has been a game-changer in Alzheimer's disease diagnosis. It helps visualize amyloid plaques in the brain, offering insights that are pivotal for initiating appropriate treatment plans. Studies reported that patients identified in earlier stages of Alzheimer's through PET imaging gained significant advantages in quality of life.
Additionally, the integration of imaging techniques into routine clinical practice supports ongoing monitoring of conditions. For example, follow-up imaging for patients recovering from strokes allows physicians to track recovery and adjust rehabilitation strategies accordingly.
In summary, case studies in brain imaging not only provide robust evidence of its applications but also demonstrate how these innovative techniques have become indispensable in enhancing our understanding and treatment of neurological and psychiatric disorders.
Challenges in Brain Imaging
Challenges in brain imaging are significant as they directly affect the interpretation and efficacy of the results derived from various imaging techniques. While these technologies have evolved remarkably, certain obstacles still hinder their overall effectiveness. Addressing these challenges can pave the way for enhanced understanding of neurological conditions and brain functions. It is crucial to recognize these limitations as they can impact both research and clinical practices.
Technical Limitations
Technical limitations play a central role in the challenges faced in brain imaging. One major issue is the resolution of images. Despite advancements in technologies like Magnetic Resonance Imaging (MRI) and Positron Emission Tomography (PET), there are thresholds beyond which further clarity can be difficult to achieve. For example, small lesions or subtle brain functions may go undetected due to limitations in imaging resolution. Additionally, how well a device can distinguish between different types of tissues or abnormalities impacts diagnostic accuracy.
Another challenge is the time it takes to acquire images. High-resolution scans often require more time, which can lead to discomfort for patients and increased costs for healthcare providers. Furthermore, factors such as patient movement during scanning can introduce artifacts that obscure critical details in images.
Lastly, not all imaging techniques capture metabolic functions effectively. For instance, EEG shows electrical activity, but it does not provide details on the underlying structures. This lack of functional specificity can limit the application of certain imaging methods for complex diagnoses.
Interpretation of Images
Interpreting brain images involves discerning useful information amid potential noise and variability. There can be a variation in images caused by differences in patient anatomy, age, or pathology, making standardized interpretations challenging. This variability can lead to diagnostic errors, especially when a definitive criterion for abnormality remains elusive.
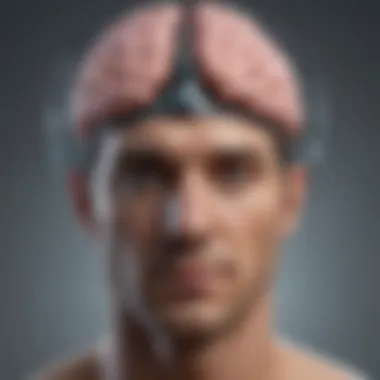
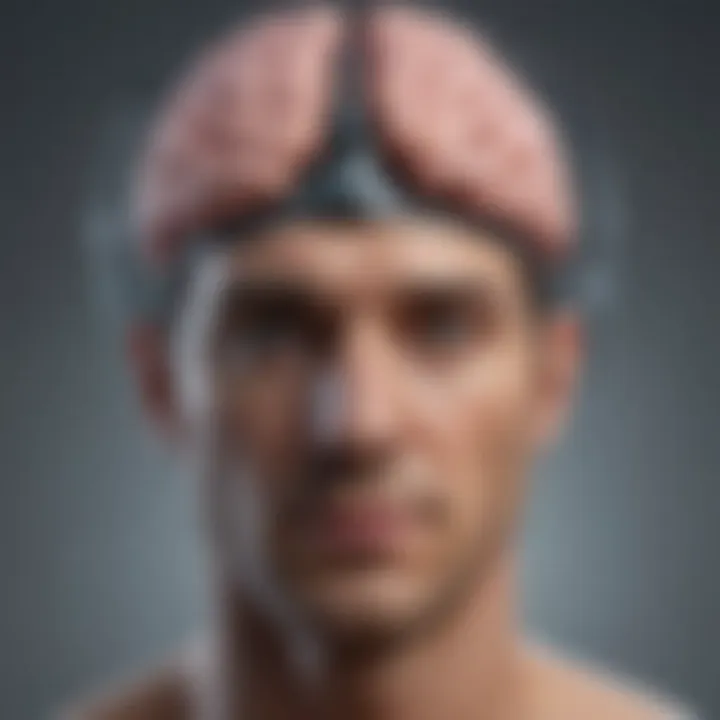
The complexity of brain structures can further complicate understanding. For example, the overlapping regions observed in MRI might not convey an accurate representation of brain networks. The subtleties in connectivity are often lost, resulting in a less comprehensive view of functional relationships among different brain regions.
Another concern is the reliance on software for quantifying brain activity or structure. The algorithms employed in processing images may vary in accuracy, leading to different interpretations. Such discrepancies underline the need for trained professionals who can critically assess images and meta-analyze findings.
"The promise of brain imaging lies in its ability to unlock the secrets of the mind, yet it is the challenges of interpretation and technical constraints that remind us of the brain's complexity."
Ethical Considerations
The ethical considerations surrounding brain imaging techniques occupy a crucial space in the discourse of neuroscience. As the field of brain imaging advances, profound implications arise concerning the treatment of sensitive data, patient autonomy, and the responsibilities of researchers. Understanding these ethical issues is essential for maintaining public trust and advancing the field responsibly.
Privacy and Data Security
Privacy concerns are paramount in brain imaging. The data generated from imaging techniques, such as fMRI scans or EEG readings, contain highly personal information that can reveal not just the structural status of the brain but its functional states and cognitive abilities. Thus, ensuring the privacy of this data is vital.
Researchers must implement robust data security measures. This includes encrypting sensitive information, limiting access to authorized personnel, and using anonymization techniques to protect subjects' identities. Additionally, institutions should establish clear protocols addressing data retention and sharing, striving for transparency with participants about how their data might be used in future research or treatment studies.
Informed Consent in Imaging Studies
Informed consent represents another cornerstone of ethical brain imaging studies. Participants should fully understand the nature of the study, including its purpose, potential risks, and benefits. Clear communication is necessary to ensure that individuals are not only aware of what they are consenting to but also that they can ask questions and withdraw from the study at any point.
In some situations, the complexity of imaging technology can hinder effective communication. Researchers must strive to simplify their explanations. This can be achieved through informative brochures, verbal briefings, and even visual aids, ensuring that participants grasp what participation entails.
Moreover, ongoing ethical reflections are needed as technology advances. Recognizing the limits of informed consent and embracing continuous education for both participants and researchers can help address these challenges.
"The challenge lies not only in the technology but also in the ethical frameworks we establish around it."
By emphasizing privacy, data security, and informed consent, the field of brain imaging can foster a more responsible and trust-driven approach, promoting not only scientific advancements but also the welfare and dignity of participants.
Future Directions in Brain Imaging
As technology evolves, the future of brain imaging holds significant promise for advancing our understanding of the human brain. This section examines critical emerging technologies and the potential for interdisciplinary approaches in brain imaging. These developments could shape the future of research, diagnostics, and treatment in neuroscience.
Emerging Technologies
New technologies in brain imaging are on the horizon. These innovations aim to enhance the resolution, speed, and accessibility of brain imaging. Techniques like hybrid imaging, integrating positron emission tomography (PET) with magnetic resonance imaging (MRI), can provide both functional and structural insights into the brain in a single session.
Another promising technology is the advancement of ultra-high field MRI scanners. Operating at higher magnetic field strengths, these scanners can capture more detailed images, allowing researchers to visualize smaller structures within the brain.
Machine learning and artificial intelligence are also playing a critical role in advancing brain imaging. These tools can aid in analyzing complex imaging data, making it easier to detect patterns and anomalies that may not be visible to the human eye.
Benefits of Emerging Technologies
- Increased Accuracy: Enhanced imaging can lead to more accurate diagnoses of brain disorders.
- Improved Treatment Planning: High-resolution images assist clinicians in crafting personalized treatment plans.
- More Accessible: With advancements, imaging techniques could become less expensive and more widely available.
As imaging technology progresses, healthcare professionals may unlock new pathways to understanding and treating neurological diseases.
Potential for Interdisciplinary Approaches
The future of brain imaging lies not just in technological advancements but also in the potential for interdisciplinary collaborations. Fields like neurobiology, cognitive psychology, and artificial intelligence are merging. This collaboration enables a more comprehensive study of brain function and behavior.
For example, combining insights from cognitive neuroscience with machine learning can lead to groundbreaking approaches that enhance diagnostic accuracy and therapeutic outcomes. Multidisciplinary teams can leverage diverse expertise to analyze complex data, yielding insights that may be missed in a single-discipline approach.
Key Aspects of Interdisciplinary Approaches
- Collaborative Research: Projects involving multiple fields can result in innovative research methodologies.
- Shared Knowledge: Diverse disciplines contribute varied perspectives, enriching understanding.
- Comprehensive Care: A cross-disciplinary approach can improve patient outcomes by integrating different treatment modalities based on comprehensive patient assessments.
Emerging technologies and interdisciplinary collaboration in brain imaging promise to unlock new opportunities for understanding the human brain. By bridging the gap between technologies and disciplines, we can pave the way for significant advancements in neuroscience, enhancing both research and clinical practices.
Finale
The realm of brain imaging has transformed our understanding of the human brain, providing insights into its complex structure and functionality. Throughout this article, we have explored various imaging techniques, highlighting their significance in clinical settings as well as in research. The importance of brain imaging cannot be overstated; it plays a pivotal role in diagnosing neurological disorders, guiding treatment decisions, and advancing our understanding of the brain's intricate workings.
Summary of Key Insights
Several key insights emerge from the discussions on brain imaging techniques. First, different methods such as Magnetic Resonance Imaging (MRI) and Positron Emission Tomography (PET) serve specific, critical functions. MRI excels in providing detailed structural images, whereas PET offers an insight into metabolic processes. Both offer complementary information that can greatly enhance our understanding of brain health and disease.
In addition, brain imaging has also shed light on cognitive processes. Functional MRI (fMRI) emerges as a particularly useful tool in cognitive neuroscience, allowing researchers to observe brain activation patterns in real time as subjects perform various tasks. This has led to discoveries about how different areas of the brain are interconnected and how they operate during specific mental activities.
Moreover, ethical considerations are essential in all imaging studies. The protection of patient data and obtaining informed consent are crucial elements that researchers must navigate carefully to ensure that studies comply with legal and ethical standards.
Final Thoughts on Future Research
Looking ahead, the future of brain imaging is promising. Emerging technologies such as advanced machine learning algorithms and hybrid imaging techniques stand to revolutionize how we understand the brain. These advancements could lead to more accurate diagnoses and personalized treatment strategies, which can enhance patient outcomes.
Furthermore, interdisciplinary approaches can offer richer insights. Collaborations between neurologists, psychologists, and data scientists can pave the way for innovative studies that delve deeper into brain function and dysfunction.